Understanding Advanced Modes of Mechanical Ventilation
Keywords
• Mechanical ventilation • Pressure ventilation • Noninvasive ventilation • Lung protective strategy • Open lung • Alveolar recruitment maneuvers
Introduction
Critical care nurses should understand the concepts of mechanical ventilation (MV), as an effective management strategy for patients requiring MV that can influence morbidity and mortality in the ICU environment. A classic study by Gajic and colleagues1 demonstrated that injudicious MV resulted in damage to the pulmonary tissue in as little as 30 minutes. This tissue injury created an alveolar inflammatory response resulting in poor lung function.1 Integrating evidence-based knowledge and skill related to MV is necessary to maximize the benefits of this intervention in the support of critically ill patients.
Approaches to optimal MV management are constantly being evaluated and refined. In the past decade, ventilators have become more sophisticated, and now can respond to patient-initiated breaths while modifying both pressure and volume in response to changing pulmonary dynamics. This allows for advanced MV modes to be utilized in the treatment of patients with complex pulmonary conditions.2 Paired with MV sophistication is a need for critical care nurses to integrate pulmonary and MV knowledge in the care of critically ill patients. The purpose of this article is to discuss the principles of MV, clinical indications, and the relevant evidence-based literature supporting lung protective strategies of MV in the care of critically ill adults. The assumption is that the reader has a working knowledge of basic MV principles (eg, pressure support [PS], continuous positive airway pressure [CPAP], assist control [AC], controlled mechanical ventilation [CMV], synchronized intermittent mandatory ventilation [SIMV], and intermittent mandatory ventilation [IMV]).
Principles and Effects of MV
Inspiratory assistance with MV completely “flips” the natural mechanics of negative pressure inspiration. MV breaths are delivered by positive pressure, thereby “pushing” air into the lungs. Pulmonary surfactant is a mixture of lipids and proteins that line the alveolus lowering surface tension and maintains alveolar surface area for effective gas exchange in the healthy lung.3 Pulmonary disease, inflammatory triggers, and the positive pressure mechanics of MV disrupt the balance of surfactant necessary to maintain open alveoli.4 Disruption in the surfactant–alveoli interface causes atelectasis that results in a ventilation/perfusion mismatch. A pulmonary shunt is described as a diffusion or perfusion impairment resulting in decreased oxygenated blood returning into arterial circulation.5
To maximize oxygenation and ventilation for the patient requiring MV, open lung strategies are implemented. Lachmann first described open lung protective therapies in 1992.6 Open lung strategy refers to employing modes of ventilation that minimize alveolar loss through positive pressure and include the recruitment of collapsed alveoli.7 This is typically achieved in MV by (1) adjusting the positive end-expiratory pressure (PEEP) to avoid alveolar collapse, (2) using a low peak inspiratory pressure (PIP) to deliver effective breaths, and (3) employing decelerating air flow patterns.4,7,8 Decelerating air flow patterns refer to the speed of the gas delivered during inspiration and the slow release of the gas, distributing air more effectively as the chest fills before expiration9 (Fig. 1). This flow pattern is considered more physiologic in nature, contributing to better alveoli gas distribution.4,7–9 Advanced MV modes that incorporate open lung strategies deliver decelerating air flow breaths predominantly using pressure-regulated modes rather than volume (ie, size of tidal volume). Newer and more sophisticated pressure modes of MV have been found to be more effective in maintaining an open lung for maximal alveolar function.4,9

Fig. 1 Decelerating flow waveform. Abbreviations: EXP, expiration; INSP, inspiration; S, seconds; V˙, flow.
(Image used by permission from Nellcor Puritan Bennett LLC, Boulder, Colorado, doing business as Covidien.)
The lung is a low-pressure system. By changing the mechanics of breathing during MV to positive pressure, intrathoracic pressures are increased; that is, more force is exerted within the thoracic space than normal. This increased intrathoracic pressure can result in symptoms of hemodynamic instability, notably reduction in cardiac output. Pushing air into the pulmonary system via MV also causes mechanical trauma to the lung, known as barotrauma. In fact, the greater the application of pressure to deliver a breath, the worse the barotrauma (ie, lung damage).8,10,11 Volutrauma, on the other hand, refers to lung damage caused by high tidal volumes. A combination of high tidal volumes and high pressures increases the stress on the pulmonary system and compound tissue injury and barotrauma.8 This tissue insult activates proinflammatory cytokines, triggering an intrapulmonary inflammatory cascade, referred to as biotrauma.8,12 Cyclic opening and closing of alveoli caused by ineffective MV exacerbates biotrauma and ventilator induced lung injury (VILI).8
Management of patients on MV attempts to provide effective oxygenation and ventilation with the least amount of pulmonary trauma. This can be accomplished through various advanced modes and settings utilized in mechanical ventilation. MV modes that employ lower tidal volumes, decelerating pressure regulated breaths, and effective PEEP are necessary to minimize VILI and are considered lung protective strategies.4,8–10
Indications for MV
Management of patients on MV requires that the critical care nurse appreciate the treatment goals for the intervention as well as the underlying pulmonary disorder(s) (ie, disease/systemic pathology, oxygenation, ventilation or mixed pulmonary disorder). Disturbances in O2 and CO2 exchange include both oxygenation and ventilation impairments: apnea, acute or impending ventilatory failure, severe hypoxemia, and respiratory muscle fatigue.13
The presence of a shunt is further appreciated when critical care clinicians calculate alveolar–arterial (A–a) gradients, shunted blood flow-to-total blood flow ratio (Qs/Qt), arterial to alveolar ratio (Pao2/PAo2), or ratio of Pao2 to fraction of inspired oxygen (Fio2) (P/F).14 Normal physiologic shunt equates to 2% to 5% of the cardiac output via the cardiac venous circulation. Shunting can be estimated noninvasively by examining P/F relationship. Clinical concerns are present when P/F are 300 mm Hg or lower. Oxygen Index (OI) calculation also incorporates the measurement of the severity of oxygenation impairment in combination with the mean airway pressure (mPaw). OI has been classically utilized in the pediatric population, but is gaining momentum in the adult population as a predictor of respiratory outcome. An OI of 20 or greater is associated with increased mortality. A P/F of 100 mm Hg or less and an OI of 30 or greater are considered measures of severe pulmonary dysfunction and a failure of conventional ventilation, which may necessitate lung protective MV modes of ventilation.15 It is clinically important to conceptualize the underlying cause and complexity of a pulmonary shunt to identify which lung protective mode of MV may be most beneficial to the patient.
MV Strategies
Traditionally, MV included principles of delivering breaths based on volume (eg, size of breath) or pressure. Volume modes would trigger inspiration and the cycling of breaths was based on programmed tidal volume, rate, and time that were believed to provide optimal ventilation. Classic strategies of ventilation (eg, CMV, AC, SIMV, IMV) were programmed irrespective of pulmonary compliance. Although these classic volume modes continue to be used in the short-term management of patients requiring MV support, they have been less effective in providing open lung protective ventilation in critically ill patients.9 Newer modes of MV based on pressure principles rather than volume provide lower tidal volumes, decelerating air flow patterns, and pressure and maintain open lung ventilation.
A large multicenter research study known as the Acute Respiratory Distress Syndrome Network study (ARDS Net) examined tidal volume (low vs high) in the treatment of patients with ARDS.15,16 This study defined low tidal volume as 4 to 8 mL/kg and high tidal volume as 12 mL/kg. Patients with ARDS and acute lung injury (ALI) were randomized to receive either high (control group) or low (treatment group) tidal volumes. The study was stopped after the enrollment of 861 patients because of decreased mortality and decreased ventilator days noted in the treatment group (ie, patients receiving low tidal volumes). Findings from this study described that lower tidal volumes protect the lungs from excessive stretch, improving patient outcomes.16
A process of incorporating lung protective strategies into clinical practice supports the use of ideal body weight (IBW) as opposed to actual or daily body weight. Tidal volume calculations based on IBW usage at 4 to 8 mL/kg, maintaining plateau pressures at 30 cm of H2O or lower and incorporating moderate levels of PEEP (ie, 5–15 cm of H2O).17 However, if alveolar derecruitment persists, as evidenced by episodes of refractory hypoxemia, careful consideration should be exercised in adjusting to higher levels of PEEP (ie, 15–24 cm H2O).14 Excessive alveolar stretch has been shown to cause barotrauma and further compromise atelectatac regions of the lung. The use of lung protective strategies in critical care has been shown to offer a survival benefit, a decrease in ventilatory days, and is currently considered the standard of care15 (Fig. 2).


Fig. 2 NIH NHLBI ARDS Clinical Network, mechanical ventilation protocol summary. Available at: http://www.ardsnet.org/system/files/Ventilator%20Protocol%20Card.pdf.
Beyond the ARDS Net study, there was a recent randomized controlled trial comparing lower tidal volume usage (4–8 mL/kg) versus historically larger tidal volumes (10–15 mL/kg) in patients who do not meet the clinical criteria for ALI. The trial was stopped prematurely because of increased rates of ALI in the higher tidal volume group.18 These findings support the usage of lower tidal volumes at 4 to 8 mL/kg, calculated from IBW for all mechanically ventilated patients.
Pressure Control Ventilation
Pressure control ventilation (PCV) is increasingly utilized in patients experiencing respiratory failure due to the ability to regulate peak inspiratory pressure, resulting in less barotrauma. Inspiratory air flow patterns are delivered in a decelerated manner in which the pressure necessary to deliver this air is decreased, resulting in a more rapid alveolar fill and equitable gas distribution. Alveoli that were previously collapsed or atelectatac are re-recruited by maintaining an increased pressure throughout the inspiratory cycle. This ventilation mode uses a predetermined inspiratory pressure that is maintained for a set period of time during inspiration. The volume of gas delivered will vary dependent on lung compliance as the tidal volume is determined by the air flow delivered according to the set pressure. For example, if the inspiratory pressure is set at 35 cm H2O to achieve a tidal volume of 400 mL, as the lungs become less compliant, the MV will continue to deliver gas at 35 cm H2O, but the tidal volume will decrease. This PCV feature reduces barotrauma but may compromise the size of tidal volume delivered. In addition to setting pressure flow parameters, inspiration and expiration time ratio (I/E ratio) can be adjusted to allow for shortened or longer phases of either of these cycles. Early ventilator modes were not sensitive to spontaneous breaths and therefore delivered pressure on inspiration, irrespective of whether the patient was initiating his own breath. Sedation or neuromuscular blockade pharmacologic agents were often administered to patients for mode tolerance. Current ventilators are more sensitive to spontaneous breathing efforts. Ventilators now sense a patient’s spontaneous breath and adjust the gas flow delivered to meet the patient’s ventilatory needs.9
The goal of PCV is to control airway pressure while meeting ventilation needs. If lung compliance decreases with worsening pulmonary disease, a decrease in tidal volume will occur. Subsequent adjustments in pressure are needed to meet the ventilatory needs of the patient while minimizing barotrauma. The most significant difference between PCV and traditional volume control modes is volume based modes deliver a set tidal volume regardless of pressure required.19 When PCV is utilized, improved gas exchange occurs, work of breathing decreases and overdistension of alveoli is reduced.20
PCV has historically been used in the management of patients with pulmonary diseases that result in poor compliance or “stiff lungs,” such as ALI, ARDS, and inhalation injury.21 Nursing considerations focus on monitoring changes in tidal volume relative to set pressure to meet ventilation and oxygenation goals. Documenting and trending tidal volumes and pressure limits, along with clinical variables such as pulse oximetry trends and arterial blood gas results are required. Initially, cardiac output may fluctuate due to changes in intrathoracic pressure. Thus, trends in blood pressure are important to monitor with increases in pressure in PCV. Because PCV mode may not synchronize with a patient’s spontaneous breathing efforts, sedation may be required.
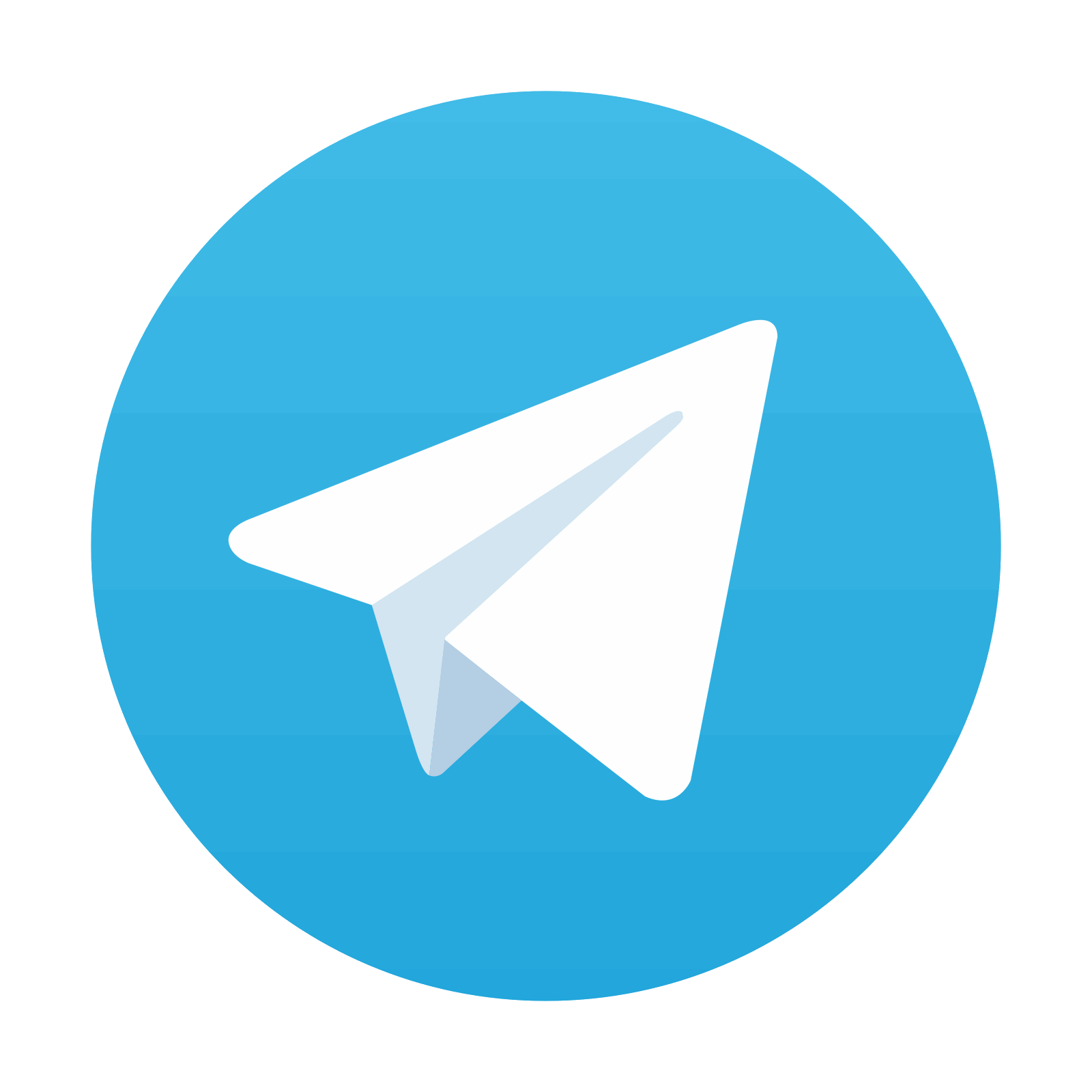
Stay updated, free articles. Join our Telegram channel

Full access? Get Clinical Tree
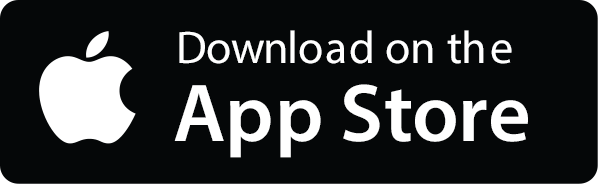
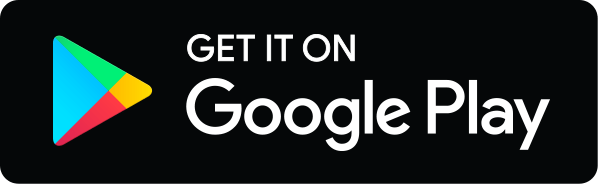