- Distribution and function of calcium and phosphate in the body
- Regulation of plasma calcium/phosphate concentrations
- The distinction between total and ionised plasma calcium and the concept of ‘adjusted’ or ‘corrected’ total plasma calcium
- Principle causes of increased and reduced plasma calcium
- Principle causes of increased and reduced plasma phosphate
- Clinical effects of abnormality in plasma calcium and phosphate concentrations
Most of the calcium and phosphate in the body is present in bone, but a small and vital fraction of each is present in blood. The two tests which are the focus of this chapter, measurement of plasma/serum concentration of calcium and phosphate, are often requested together with two other blood tests considered in Chapter 11: albumin and alkaline phosphatase. The four tests, together known as a bone profile, are used in the first line investigation of patients who are suspected of suffering metabolic bone disease. The origins of bone disease often lie in distant organs (parathyroid glands, kidneys and gastrointestinal tract) that are involved in the regulation of plasma calcium and phosphate concentration. Measurement of calcium and phosphate is thus often appropriate in investigation of patients suffering disease of these organs. Three other broad groups of patients in whom plasma calcium is often measured are those suffering malignant disease, the critically ill and preterm babies.
Point of care testing in intensive care units often includes the measurement of plasma calcium. In these circumstances nursing staff may be responsible for the analytical process.
Normal physiology
Dietary source of calcium (Ca) and phosphorus (P)
A normal western diet contains around 1000 mg Ca/day. Recommended minimum intake for adults is 700 mg/day. The most common source is milk and dairy products; alternative sources include green leafy vegetables, soybean and nuts. Flour and some breakfast cereals are fortified with calcium, and in some areas tap water is a significant source of calcium. Less than half of ingested calcium is normally absorbed from the gastrointestinal tract (small intestine) the rest is excreted in faeces. The actual amount that is absorbed is under hormonal control and so is adjusted to suit requirements at the time.
Most foods contain some phosphorus and a normal diet contains around 1000 mg/day, predominantly from meat and dairy foods.
Body distribution of calcium and phosphate
The adult body contains around 1 kg of Ca and 600 g of P, the latter combined with oxygen and present as phosphate (PO4). Almost all (99%) of this calcium and 85% of phosphate is contained in bone as hydroxyapatite crystals, which has the chemical formula Ca10(PO4)6OH2. Together with the protein collagen, hydroxyapatite crystals are the major structural components of bone and teeth; calcium and phosphate together comprise 65% of bone weight. A small amount (<1%) of the calcium in bone is exchangeable with calcium in blood, this allows movement of calcium into bone when blood concentration is high and in the reverse direction when blood calcium is low.
Just 350 mg (8.7 mmol) of calcium circulates in blood plasma at a concentration of around 2.5 mmol/L. Half of this is bound to the protein albumin and is physiologically inert, the rest circulates as ‘free’ or ionised calcium (Ca++); it is only this fraction that is physiologically active and therefore of clinical significance. Finally, there is a very tiny but physiologically vital fraction of total body calcium that resides in all cells. The concentration of calcium in cells is of the order of nanomol/L, one thousandth of the concentration in blood plasma.
The 15% of phosphate which is not present in bone is divided between tissue cells and the extracellular compartment, which includes blood plasma. Around 1% of the body’s phosphate is present in blood plasma at a concentration of around 1.0 mmol/L.
Function of calcium and phosphate
Both calcium and phosphate have significant roles other than the one they share as major structural component of bones and teeth. The ionised calcium that circulates in blood plasma is an essential cofactor for the enzymes involved in blood coagulation. It is also a source of the calcium required for many cellular processes, including electrical conductance of nerve impulses, cardiac and skeletal muscle contraction and the signalling between nerve and muscle cells (neuro-muscular transmission). Calcium signalling is an essential part of the process by which many hormones affect target tissue cells. Thus the function of many hormones is dependent on ionised calcium. There are in fact few physiological functions that can proceed in the absence of minute quantities of intracellular ionised calcium.
Phosphate is an integral part of many key biological molecules. These include the nucleic acids DNA and RNA, phospholipids (structural components of all cell membranes) and many other substances of intermediary cell metabolism, including adenosine triphosphate (ATP), the most significant source of chemical energy for cell metabolism. Inorganic phosphate, that is the phosphate which is not integrated into larger biological molecules, acts as a buffer in blood and urine, and is thus involved in the vital process of maintaining normal blood pH.
The many functions of calcium and phosphate depend on the maintenance of their plasma concentrations within narrow limits. The next section describes the mechanisms that maintain normal plasma calcium concentration. Disturbance of one or more of these mechanisms is often the cause of abnormal plasma calcium and/or phosphate results.
Normal control of plasma calcium and phosphate concentrations
The concentration of calcium in plasma reflects a balance between dietary derived calcium absorbed via the gastrointestinal tract and that lost from the body in urine. In addition, as outlined above, calcium can move between plasma and bone. These routes of calcium movement, and thereby plasma calcium concentration, are under the control of two hormones: parathormone (PTH) and the vitamin D derived hormone, calcitriol. These hormones also have effect on plasma phosphate concentration.
Source, secretion and effect of PTH
PTH is the calcium regulating hormone produced and secreted by the four tiny (rice grain sized) parathyroid glands, sited close to, or embedded in the surface of, the thyroid gland. By virtue of a protein called the calcium sensing receptor (CaSR) located in the membrane of parathyroid cells, the parathyroid glands are able to sense ionised calcium concentration of blood flowing through the gland and respond to changes in concentration. Reduction in concentration of plasma ionised calcium stimulates parathyroid gland production and secretion of PTH (Figure 6.1). The PTH is transported in blood to its two target organs: kidney and bone. The effect of PTH is to release calcium from bone to blood and decrease renal excretion of calcium in urine. The net result is restoration of normal plasma ionised calcium concentration. As plasma ionised calcium concentration rises towards normal, PTH secretion diminishes. PTH also has a plasma phosphate lowering effect through its action on kidney, where it increases urine excretion of phosphate. Overall then the effect of PTH is to raise plasma ionised calcium and lower plasma phosphate.
Source, secretion and effect of calcitriol
Calcitriol (alternative name 1,25 dihydroxycholecalciferol), the other main calcium regulating hormone, is derived from vitamin D and released to blood from the kidneys.
Although diet is a source of vitamin D, it is also synthesised in the skin by the action of sunlight on a cholesterol-like substance (7-dehydrocholesterol) present in skin cells. By a two step process vitamin D derived from diet, as well as that synthesised in skin, is converted to calcitriol (Figure 6.2). The first step occurs in the liver and the second in the kidneys. The second step, which results in release of calcitriol to blood from kidney, is under the control of PTH. When plasma calcium is low and therefore PTH levels are high, renal production and secretion of calcitriol is also high. Production and secretion of calcitriol is like that of PTH promoted by reduced plasma ionised calcium and inhibited by rising plasma ionised calcium concentration as well as rising plasma phosphate concentration.
The principle action of calcitriol is on the gastrointestinal tract where it promotes absorption of dietary calcium and phosphate. The net effect is a rise in plasma calcium and phosphate concentrations. By the integrated action of PTH and calcitriol, plasma calcium and phosphate concentrations are maintained within normal limits.
To summarise, the maintenance of normal plasma calcium and phosphate concentration depends on:
- Normal diet containing adequate calcium, phosphorus and vitamin D.
- Normal gastrointestinal function for dietary absorption of all three.
- Exposure to sunlight for adequate endogenous production of vitamin D.
- Normal parathyroid function: for appropriate secretion of PTH.
- Normal liver and renal function: for conversion of vitamin D to calcitriol.
- Normal renal function: for secretion of calcitriol and appropriate adjustment of calcium and phosphate loss in urine.
- Normal bone metabolism for appropriate movement of calcium (and phosphate) between blood and bone.
The preservation of normal plasma ionised calcium concentration is more important for survival than preserving normal amounts of calcium in bone and, if calcium is in short supply, the body sacrifices bone mineralisation in order to maintain plasma ionised calcium concentration.
Measurement of calcium and phosphate
The concept of ‘corrected’ or ‘adjusted’ total calcium
As already discussed, the calcium present in blood plasma comprises two almost equal fractions: half is bound to the protein albumin and the rest is unbound free, ionised calcium (Ca++). Ideally, the physiologically active, clinically important ionised calcium fraction would be measured, but for reasons discussed below it is more convenient and cheaper to measure total plasma calcium (i.e. the calcium bound to albumin plus unbound (free) ionised calcium), and it is total plasma calcium concentration that is routinely measured in the clinical laboratory. The validity of measuring total plasma calcium as a proxy for measuring plasma ionised calcium depends crucially on the concentration of calcium bound to albumin remaining constant in both health and disease, then any change in total plasma calcium concentration can only be due to change in plasma ionised calcium concentration.
In practice, so long as the amount of albumin in plasma (i.e. plasma albumin concentration) is constant then the amount of calcium bound to albumin is constant and total calcium concentration accurately reflects plasma ionised calcium concentration. However, if plasma albumin concentration is abnormal then total plasma calcium is abnormal, providing the false impression that plasma ionised calcium is also abnormal.
To overcome this potential problem it is important to take account of plasma albumin concentration when interpreting plasma total calcium concentration. If plasma albumin is either abnormally high or abnormally low a correction must be made to the total plasma calcium concentration. Various formulae have been devised to make the correction – this is one of the most commonly used:
If plasma albumin is greater than 45 g/L
‘corrected’ or ‘adjusted’ total Ca (mmol/L) = measured total Ca (mmol/L) – [0.02 × albumin (g/L) – 45].
If plasma albumin is less than 40 g/L
‘corrected’ or ‘adjusted’ total Ca (mmol/L) = measured Ca (mmol/L) + [0.02 × (40 – albumin g/L)].
In essence the ‘corrected’ or ‘adjusted’ total calcium is the total calcium value that would be obtained if the plasma albumin concentration were normal.
Although historically not necessarily the case, most laboratories now have a policy of measuring plasma albumin on all samples submitted for total plasma calcium measurement and automatically reporting a ‘corrected’ or ‘adjusted’ total calcium result. If this is not the case then a request for plasma albumin must be made with plasma calcium requests if it is thought that plasma albumin may be abnormal.
Measurement of plasma ionised calcium
For some patient groups, most notably the critically ill, the formulae used to correct total calcium are invalid1 and for these patients it is important to measure plasma ionised calcium rather than plasma total calcium. Point of care analysers, including blood gas analysers sited in intensive care units, recovery rooms and emergency departments, usually have the capacity for measurement of plasma ionised calcium. Clinical laboratories may or may not have the capacity to measure plasma ionised calcium but there must be good clinical justification for eschewing request for total plasma calcium estimation in favour of a request for plasma ionised calcium estimation.
Patient preparation
No particular patient preparation is necessary.
Timing of sample
Blood for both calcium and phosphate can be collected at any time of day.
Sample requirements
For laboratory measurement of total plasma calcium
Around 5 ml of venous blood is sufficient for laboratory estimation of calcium and phosphate. Measurement may be made on either plasma or serum recovered from a blood sample. If local policy is to use serum then blood must be collected into a plain collection tube that does not contain anticoagulant. Plasma estimation requires collection into a tube containing the anticoagulant lithium heparin. No other anticoagulant is suitable for calcium and phosphate analysis.
Use of a tourniquet can affect calcium results. Blood for calcium estimation should if possible be collected without the use of a tourniquet. Poor technique during blood collection can cause damage to the membrane of blood cells and resulting haemolysis. Phosphate is present at much higher concentration in the cells of blood than plasma and damage to cells causes release of phosphate to plasma and a falsely raised result. Haemolysed samples are thus unsuitable for phosphate estimation.
For point of care measurement of ionised calcium
Plasma ionised calcium is usually measured at the point of care using the same anticoagulated arterial blood specimen used for blood gas analysis. The same specialised attention to sampling and handling of blood for blood gases is necessary if only ionised calcium estimation is required. The exacting requirements for sample collection and handling is a major reason why ionised calcium measurement is not suited to routine ‘high throughput’ measurement in the laboratory.
Ionised calcium can be measured on venous as well as arterial blood, provided it is processed in the same way arterial blood is processed for blood gas analysis. Phosphate cannot be measured at the point of care – only laboratory based methods are available.
Interpretation of results
Effect of blood pH on calcium
The proportion of total calcium in blood that is in the ionised ‘free’ state is affected by the pH of blood. High blood pH (alkalosis) results in less calcium being in the ionised state and low blood pH (acidosis) results in more calcium being in the ionised state. The clinical implication of this effect of blood pH on plasma total calcium is demonstrated in Case history 11, but the general point to be made here is that interpretation of total calcium results, but not plasma ionised calcium results, is a little more complicated if the patient has a condition in which acid-base balance is disturbed.
Approximate reference (normal) ranges
Plasma/serum calcium (‘corrected’ total) | 2.20–2.60 mmol/L |
Plasma ionised calcium | 1.15–1.30 mmol/L |
Plasma/serum phosphate | 0.80–1.40 mmol/L |
Critical values
Plasma/serum calcium (‘corrected’ total) | <1.50 mmol/L >3.30 mmol/L |
Plasma calcium (ionised) | <0.80 mmol/L >1.60 mmol/L |
Terms used in interpretation
Hypercalcaemia | increased amount of calcium in blood, that is plasma/serum calcium (‘corrected’ total) > 2.60 mmol/L or plasma ionised calcium >1.30 mmol/L. |
Hypocalcaemia | decreased amount of calcium in blood, that is plasma/serum calcium (‘corrected’ total) <2.20 mmol/L or plasma ionised calcium <1.15 mmol/L. |
Hyperphosphataemia | increased amount of phosphate in blood, that is plasma/serum phosphate >1.40 mmol/L. |
Hypophosphataemia | decreased amount of phosphate in blood, that is plasma/serum phosphate < 0.80 mmol/L. |
Causes of raised plasma/serum calcium
The two most common causes of hypercalcaemia are malignant disease (cancer) and primary hyperparathyroidism.
Hypercalcaemia and malignant disease
Excessive production by tumour cells of a protein called parathormone-related polypeptide (PTHrP) is thought to be the main cause of the hypercalcaemia that can affect cancer patients2. As its name implies, PTHrP is structurally and functionally similar to the calcium regulating hormone parathormone (PTH) produced by the parathyroid glands. The action of PTHrP is like that of PTH to promote calcium movement from bone to blood, with a consequent rise in plasma calcium concentration. However whereas PTH production is controlled by plasma calcium concentration, that is promoted by reduced calcium concentration and inhibited by increased concentration, PTHrP production by tumour cells is under no such control. The inevitable consequence of the uncontrolled PTHrP production by tumour cells is abnormal loss of calcium from bone and increasing blood calcium concentration.
Although hypercalcaemia can occur in all cancer types, it is more common in some than others, so that cancers of the lung, breast, head, throat and oesophagus are more likely to be associated with hypercalcaemia than those of the kidney, bowel and stomach.
Multiple myeloma, a haematological malignancy of the bone marrow is the malignant disease most commonly associated with hypercalcaemia; nearly a half of all myeloma patients are affected. In this case hypercalcaemia is not due primarily to PTHrP, but to the bone destruction (and consequent release of calcium) that results from infiltrating tumour cells.
Generally speaking hypercalcaemia develops late in the evolution of solid tumour cancers, when disease is at an advanced stage and has spread beyond the primary site particularly to bone; it is therefore a poor prognostic sign. Still it is important to detect because treatment is invariably successful in normalising plasma calcium and relief from symptoms of hypercalcaemia can improve the quality of life of cancer patients.
Primary hyperparathyroidism
Persistently raised plasma calcium in the absence of malignant disease is most likely due to primary hyperparathyroidism, the second most common cause of hypercalcaemia among hospitalised patients and the most common cause among the general population. Although this condition can occur at any age and in both sexes, it most commonly affects post-menopausal women. Excessive, uncontrolled secretion of PTH by a benign tumour, called an adenoma, in one of the four parathyroid glands is the cause of the hypercalcaemia in nearly all cases of primary hyperparathyroidism. In a minority of patients increased PTH is a result of an abnormal increase in size (hyperplasia) of all parathyroid glands.
Many cases are discovered by chance when biochemical screening for investigation of apparently unrelated symptoms reveals raised plasma calcium concentration. Non-specific symptoms of hypercalcaemia (e.g. depression, constipation) may have been present for years before diagnosis and some patients (usually those with only very mild hypercalcaemia, in the range 2.60–2.80 mmol/L) are asymptomatic. If left untreated primary hyperparathyroidism can cause bone demineralisation (due to the uncontrolled effect of PTH on bone), deposition of calcium in kidney and consequent renal disease, urinary calcium stones and, in rare cases, hypercalcaemia is of such severity that life is threatened3. Surgical removal of the offending adenoma is the only curative treatment.
Rarer causes
The two causes outlined above account for close to 80% of hypercalcaemia cases. Relatively common conditions that are only rarely associated with hypercalcaemia include chronic kidney disease and thyrotoxicosis. Around 10% of patients suffering sarcoidosis, a rare chronic disease predominantly affecting the lungs, are hypercalcaemic because of abnormal increase in calcitriol production and consequent increased absorption of dietary calcium. The same mechanism accounts for the hypercalcaemia that can affect those with tuberculosis (TB) and those who have taken excessive doses of vitamin D. Some drugs, notably thiazide diuretics and lithium, can cause hypercalcaemia. Severe hypercalcaemia is a feature of a life-threatening condition called milk-alkali syndrome4 caused by excessive use of over the counter medicines (e.g. Rennie tablets) that contain calcium carbonate. The incidence of milk-alkali syndrome (sometimes called calcium-alkali syndrome) has been increasing in recent years and it is now considered a relatively common cause of severe hypercalcaemia. Occasionally no cause can be identified for hypercalcaemia.
The main causes of increased plasma/serum calcium are summarised in Table 6.1
Causes of reduced plasma/serum calcium
Hypocalcaemia is less common than hypercalcaemia. Since the action of calcium regulating hormones, PTH and calcitriol, is to raise plasma calcium, it is to be expected that deficiency or reduced action of either hormone may lead to hypocalcaemia.
Table 6.1 Principal causes of abnormal plasma calcium and phosphate.
Causes of increased plasma calcium (hypercalcaemia) |
|
Causes of decreased plasma calcium (hypocalcaemia) |
|
Causes of increased plasma phosphate (hyperphosphataemia) |
|
Causes of decreased plasma phosphate (hypophosphataemia) |
|
Hypoparathyroidism – reduced production of PTH
Hypoparathyroidism is rare, most cases being the result of damage to parathyroid glands during surgery. The anatomical intimacy of parathyroid and thyroid render the parathyroid glands and/or their blood supply particularly vulnerable to unintended damage during thyroid surgery. The small size and variable anatomy of parathyroid glands contributes to this vulnerability. The same mechanism accounts for the hypocalcaemia which may develop after surgical removal of parathyroid adenoma, to cure primary hyperparathyroidism.
Around 10% of patients undergoing total thyroidectomy develop temporary hypoparathyroidism. Permanent hypoparathyroidism is much less common, occurring in just 4% of patients5. The risk of hypoparathyroidism and consequent hypocalcaemia is reduced by less radical surgery (partial rather than total thyroidectomy), but the post operative management of all patients recovering from thyroid and parathyroid surgery includes careful monitoring of plasma calcium.
Rarer causes of hypoparathyroidism and consequent hypocalcaemia include damage to the parathyroid as a result of autoimmune disease and congenital absence or reduced development of the parathyroid glands.
Reduced production of calcitriol
Hypocalcaemia is a feature of the childhood bone disease rickets and its adult equivalent, osteomalacia. In both cases hypocalcaemia is due to deficiency of vitamin D, the substance from which calcitriol is synthesised. Hypocalcaemia develops because in the absence of adequate calcitriol, normal amounts of dietary calcium and phosphate cannot be absorbed. Deficiency of vitamin D that leads to hypocalcaemia can arise in a number of ways. It may simply be because diet contains insufficient vitamin D or because disease of the gastrointestinal tract (e.g. coeliac, Crohn’s disease) or pancreas (chronic pancreatitis) prevents normal amounts of vitamin D being absorbed from food. Lack of exposure to sunshine and consequent reduced synthesis of vitamin D can also cause vitamin D deficiency.
The normal physiological response to reduced plasma calcium, whatever its cause, is increased production of PTH and resulting movement of calcium from bone to blood. If vitamin D deficiency remains uncorrected, PTH secretion remains high. It is the loss of calcium from bone induced by PTH that leads to the bone deforming features of rickets in children and bone demineralisation of osteomalacia in adults.
Hypocalcaemia is a common feature of CKD and a less common feature of some chronic liver disorders and primary biliary cirrhosis. This reflects the key roles that both kidney and liver play in conversion of vitamin D to calcitriol, as well as the specific role that the kidneys play in minimising calcium loss in urine. The hypocalcaemia associated with chronic liver disease is potentiated by vitamin D deficiency, consequent on reduced bile production by the liver (bile acids are required for absorption of vitamin D). Some anticonvulsant drugs, which are metabolised in the liver, reduce vitamin D metabolism in the liver and thereby calcitriol production. Patients taking these drugs are at long-term risk of hypocalcaemia and consequent bone demineralisation.
Hypocalcaemia in the critically ill
Hypocalcaemia is a common feature of critical illness. Up to 85% of patients being cared for in intensive care units develop hypocalcaemia6. Only methods that measure ionised calcium should be used to assess calcium status among these patients (already discussed). The conditions most often associated with hypocalcaemia among intensive care patients include: acute kidney injury, alkalosis, sepsis, acute pancreatitis, severe burns and rhabdomyolysis. Massive blood transfusion may cause hypocalcaemia.
Neonatal hypocalcaemia
Hypocalcaemia is not uncommon during the first day or two of life. Foetal bone development requires a relatively high plasma calcium concentration in utero. The physiological transition from an intrauterine environment to neonatal independence includes a rapid reduction in plasma calcium during the first 24–48 hours of life. Transient hypocalcaemia during this early period is thought to be an exaggeration of this physiological response due to an insufficient PTH response from immature parathyroid glands (transient hypoparathyroidism). Premature and low birth weight babies being cared for in intensive care are at particular risk of this hypocalcaemic mechanism, as are babies born to diabetic mothers.
Late onset neonatal hypocalcaemia, occurring during the second week after birth is thought be the result of inadequate renal response to PTH due to immature kidneys. Vitamin D deficiency, as a result of maternal deficiency during pregnancy, may also manifest as hypocalcaemia during this early period of life.
The main causes of reduced plasma/serum calcium are summarised in Table 6.1.
Causes of raised plasma/serum phosphate
By regulating the amount of phosphate that is lost from the body in urine, the kidneys have a central role in maintaining normal blood levels. The most common cause of raised plasma/serum phosphate is CKD. It is also a feature of AKI. A failing kidney is unable to excrete excess phosphate as efficiently as normal, so blood concentration rises.
Excessive intake of phosphate is a rare cause of hyperphosphataemia. This may occur in patients who are being fed parenterally. Vitamin D enhances absorption of dietary phosphate so that increased serum phosphate may occur in cases of vitamin D intoxication.
The very high concentration of phosphate within tissue cells as compared with that of extracellular fluid (blood) means that in cases of severe tissue destruction (e.g. rhabdomyolysis) there is an increase in blood levels as phosphate leaks from damaged cells to blood. Any illness or treatment (e.g. cancer chemotherapy) which is associated with marked tissue catabolism can result in raised plasma phosphate.
As already discussed, PTH has an important role in regulating plasma phosphate levels – it increases renal excretion of phosphate. This explains the raised plasma phosphate that is evident in those with PTH deficiency (hypoparathyroidism).
The main causes of increased plasma/serum phosphate are summarised in Table 6.1.
Causes of reduced plasma/serum phosphate
Hypophosphataemia develops as a result of three main mechanisms: reduced phosphate entering the body, increased phosphate losses (in urine) from the body and movement of phosphate from blood plasma into cells.
The presence of phosphate in almost all foodstuffs makes inadequate dietary intake a rare cause of hypophosphataemia, but it can occur if poor nutrition is long standing, for example, in chronic alcoholism and eating disorders (e.g. anorexia nervosa). Inadequate absorption of phosphate may lead to hypophosphataemia in patients with chronic gastrointestinal conditions such as Crohn’s disease and coeliac disease; this being part of a wider malabsorption syndrome involving many dietary nutrients. Calcitriol, the hormone derived from vitamin D, is essential for adequate absorption of dietary phosphate so that vitamin D deficiency leads to hypophosphataemia.
One of the actions of PTH is to increase phosphate excretion in urine. It is to be expected then that PTH excess (hyperparathyroidism) is associated with excessive losses of phosphate in urine and consequent hypophosphataemia.
Hypophosphataemia caused by movement of phosphate from blood into tissue cells is a feature of diabetic ketoacidosis and respiratory alkalosis, which is discussed in Chapter 7.
The main causes of reduced plasma/serum phosphate are summarised in Table 6.1.
Consequences of abnormal plasma/serum calcium and phosphate
Many of the clinical consequences of abnormal plasma calcium can be related to the central role that calcium plays in transmission of signal between nerve cells (neural transmission) and between nerve and muscle cells (neuromuscular transmission). The function of central nervous system, skeletal muscle, heart and gastrointestinal tract are particularly dependant on this calcium mediated signalling and any or all of these organ systems may be affected if plasma calcium is abnormal.
Signs, symptoms and consequences of raised calcium
In general, the range and severity of symptoms reflect the degree of hypercalcaemia. So that many patients with mild hypercalcaemia, usually defined as plasma/serum calcium between 2.60 and 3.00 mmol/L are asymptomatic, whilst those with calcium greater than 3.50 mmol/L almost always manifest a range of signs and symptoms, some of which threaten survival.
Common gastrointestinal symptoms include nausea, vomiting and constipation. Central nervous system involvement can result in neuro-psychiatric symptoms including lethargy, depression and confusion; psychosis, seizures and coma may occur. Muscular weakness and fatigue are common. Cardiac involvement includes arrhythmias with characteristic ECG changes. Cardiac arrest can be precipitated by severe hypercalcaemia, so that a plasma total calcium concentration greater than 3.5 mmol/L constitutes a clinical emergency, warranting immediate calcium lowering therapy.
Inability of the kidneys to concentrate urine effectively is a common feature of moderately severe hypercalcaemia, this is manifest as polyuria (increased urine volume) and resulting polydipsia (thirst). Long standing hypercalcaemia, even if it is mild, can lead to deposition of calcium in kidneys and tendency to form renal stones; increasing loss of renal function consequent on either of these can lead in the long term to CKD.
Signs, symptoms and consequences of reduced calcium
Mild hypocalcaemia, roughly defined as total corrected plasma calcium in the range 1.80–2.2 mmol/L, may occur without symptoms, but more severe hypocalcaemia is invariably associated with symptoms of tetany that result from increased neuro-muscular excitability. These include loss of nerve sensation, tingling sensation, painful muscular spasms, twitching and, in severe cases, convulsions and seizures. Laryngeal spasm restricts normal respiration and can be a life-threatening effect of severe hypocalcaemia.
Cardiac arrhythmias with characteristic ECG changes may be a feature. Central nervous system involvement may result in neuropsychiatric symptoms such as anxiety, depression and psychosis among those with long-standing hypocalcaemia. Long-standing hypocalcaemia is associated with higher than normal risk of cataracts and heart failure.
Signs, symptoms and consequences of raised phosphate
There are no symptoms directly attributable to a raised plasma phosphate but by the combined action of several mechanisms, raised phosphate causes a reduction in plasma/serum calcium. For this reason many patients with hyperphosphataemia may be suffering symptoms of hypocalcaemia (outlined already). In the long term, hyperphosphataemia can lead to the precipitation of calcium phosphate in tissues, a pathological process known as calcification. One important aspect of this is that calcification of arteries is involved in the process of atherosclerosis, which leads to coronary heart disease and strokes. It is now suspected that the high risk of a cardiovascular death in patients with CKD is due, at least in part, to the hyperphosphataemia and resulting calcification of arteries that so often occurs in this patient group7.
Signs, symptoms and consequences of reduced phosphate
Most patients with hypophosphataemia have plasma phosphate in the range 0.5–0.8 mmol/L. Such a mild reduction is not associated with symptoms and is of little clinical significance. However severe hypophosphataemia, usually defined as plasma/serum phosphate <0.3 mmo/L, has important clinical consequence. Symptoms include muscle weakness: this may affect muscles involved in respiration, causing respiratory difficulties. Severe muscle destruction (rhabdomyolysis), consequent on reduced ATP, can occur. Reduced phosphate in erythrocytes can cause increased red cell destruction and resulting anaemia. Central nervous system symptoms include confusion, seizures and rarely coma.
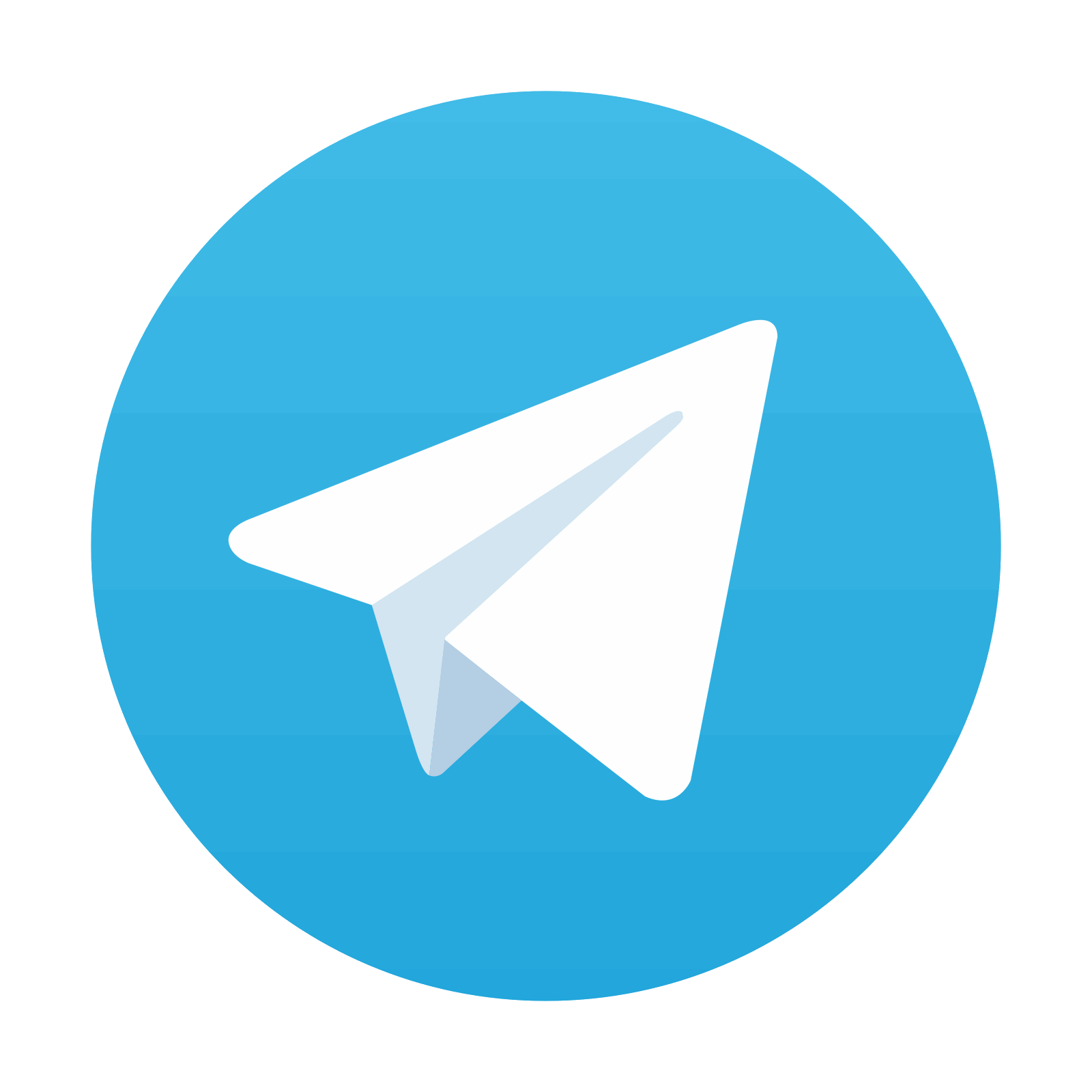
Stay updated, free articles. Join our Telegram channel

Full access? Get Clinical Tree
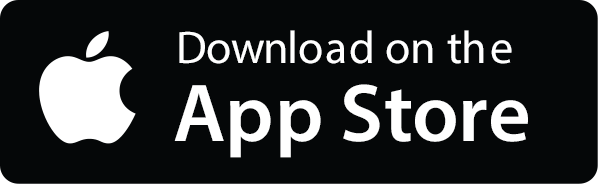
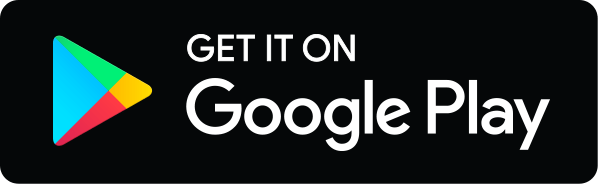