CHAPTER 37. Endocrine Emergencies
Colette M. Morey
The endocrine system is an integrated complex of hormone-secreting glands. This system is instrumental in regulating metabolism, tissue function, growth and development, moods and emotions, and works to maintain homeostasis in response to physiologic stress. 8 Dysfunction of one endocrine gland can affect the physiology of the entire body. Without prompt assessment, identification, and management, endocrine disturbances may result in life-threatening consequences. The majority of endocrine emergencies encountered in the emergency department (ED) are related to diabetes. This chapter addresses selected disorders related to diabetes, pituitary, thyroid, and adrenal pathology, as well as alcoholic ketoacidosis, a metabolic emergency.
ENDOCRINE SYSTEM PHYSIOLOGY
The endocrine system consists of the hypothalamus, pituitary, thyroid, parathyroids, adrenals, pancreas, testes, and ovaries (Figure 37-1). Each gland produces and stores one or more hormones that have specific, unique functions. Table 37-1 identifies the hormones produced by the major endocrine glands, their target tissue, and their functions. Activities of the testes and ovaries are discussed in Chapter 35 and Chapter 43.
![]() |
FIGURE 37-1 Location of the major endocrine glands. Parathyroid glands actually lie on the posterior surface of the thyroid. (From Lewis SL, Heitkemper MM, Dirksen SR: Medical-surgical nursing: assessment and management of clinical problems, ed 7, St. Louis, 2007, Mosby.) |
Hormones | Target Tissue | Functions |
---|---|---|
ANTERIOR PITUITARY (ADENOHYPOPHYSIS) | ||
Thyroid-stimulating hormone (TSH) or thyrotropin | Thyroid gland | Stimulates synthesis and release of thyroid hormones, growth and function of thyroid. |
Adrenocorticotropic hormone (ACTH) or corticotrophin | Adrenal cortex | Fosters growth of adrenal cortex; stimulates secretion of glucocorticoids. |
POSTERIOR PITUITARY (NEUROHYPOPHYSIS) | ||
Antidiuretic hormone (ADH) or vasopressin | Renal tubules, vascular smooth muscle | Promotes reabsorption of water. Raises blood pressure by inducing moderate vasoconstriction. |
THYROID | ||
Thyroxine (T 4) | All body tissues | Precursor to T 3. |
Triiodothyronine (T 3) | All body tissues | Regulates metabolic rate of all cells and processes of cell growth and tissue differentiation. |
ADRENAL MEDULLA | ||
Epinephrine (adrenalin) | α- and β-adrenergic receptors | Increases heart rate and stroke volume, dilates pupils, constricts arterioles in the skin and digestive system, dilates arterioles in skeletal muscles. Relaxes bronchial smooth muscles. Triggers the release of glucose from energy stores. |
Norepinephrine | α- and β-adrenergic receptors | Effects similar to epinephrine although longer acting. Also increased alertness and arousal. |
ADRENAL CORTEX | ||
Corticosteroids (e.g., cortisol, hydrocortisone) | All body tissues | Maintains blood glucose, suppresses the immune response, and is released as part of the body’s response to stress. |
Mineralocorticoids (e.g., aldosterone) | Kidney | Regulates sodium and potassium balance and thus water balance. |
PANCREAS | ||
Insulin (from beta cells) | General | Promotes movement of glucose out of blood and into cells. |
Glucagon (from alpha cells) | General | Promotes movement of glucose from storage and into blood. |
Hormone activity is the result of feedback loops, nerve stimulation, and intrinsic rhythms. Renal and liver function and external factors such as pain, stress, or fear also affect hormone release. Excessive amounts of circulating hormones inhibit hormone release, whereas low levels lead to increased hormone release. Neural stimulation triggers increased glandular activity and release of hormones. Intrinsic rhythms vary from hours to weeks and provide another method of hormone control.
Hypothalamus
The hypothalamus creates part of the walls and floor of the third ventricle. Various centers in the anterior and posterior hypothalamus control most endocrine functions and many emotional behaviors. Nerve tracts from the hypothalamus join the posterior pituitary, which lies just below. Posterior pituitary hormones are actually synthesized in the hypothalamus and then transferred along axons for storage in the posterior pituitary. The hypothalamus regulates anterior pituitary action by inhibiting or releasing certain hormones.
Pituitary
The pituitary gland, approximately 1 cm in all directions, lies within the sella turcica of the middle cranial fossa. Two physiologically distinct areas are found in the pituitary. The anterior pituitary contains secretory cells, whereas the posterior pituitary consists of neural cells that serve as a supporting structure for nerve fibers and nerve endings. Hormones secreted by the anterior pituitary include growth hormone, adrenocorticotropic hormone (ACTH), thyroid-stimulating hormone (TSH), prolactin, follicle-stimulating hormone, and luteinizing hormone. Antidiuretic hormone (ADH) and oxytocin are secreted by the posterior pituitary.
Thyroid
The thyroid gland consists of two lobes connected by an isthmus. This butterfly-shaped gland in the anterior neck below the cricoid cartilage partially surrounds the trachea. Thyroid hormone release is regulated through a complex feedback system between the hypothalamus and anterior pituitary. This main metabolic regulator contains follicular cells that secrete thyroxine (T 4) and triiodothyronine (T 3) in response to stimulation by the pituitary. Calcitonin originating in parafollicular cells in the thyroid affects calcium metabolism.
Parathyroids
The parathyroid glands are four or more small glands (the size and shape of a grain of rice) located on the posterior surface of the thyroid gland. The sole function of these glands is to maintain the body’s calcium level within a very narrow range so that the nervous and muscular systems can function normally. When serum calcium levels drop, calcium-sensing receptors in the parathyroid gland are activated to release parathyroid hormone (PTH). PTH increases blood calcium levels by stimulating osteoclasts to break down bone and release calcium. It also increases gastrointestinal absorption of calcium by activating vitamin D and promotes calcium reabsorption by the kidneys.
Adrenals
The adrenal glands, located in the retroperitoneal area above the upper pole of each kidney, consist of an outer cortical layer and inner medullary layer. The adrenal cortex produces mineralocorticoids (e.g., aldosterone), glucocorticoids (e.g., cortisol), and androgens. Aldosterone is critical for maintaining internal fluid balance, whereas glucocorticoids are major players in the body’s ability to resist stress. The adrenal medulla releases the catecholamines epinephrine and norepinephrine in response to sympathetic stimulation.
Pancreas
The pancreas is situated behind the stomach in the retroperitoneal space. The body of the pancreas extends horizontally across the abdominal wall with the head in the curve of the abdomen and the tail touching the spleen. Exocrine and endocrine cells are found in the pancreas. Acini are exocrine cells that release amylase, lipase, and other enzymes that aid in digestion. Three types of endocrine cells within the islet of Langerhans produce hormones that regulate serum glucose levels. Alpha cells secrete glucagon, beta cells produce insulin, and delta cells secrete somatostatin, which inhibits glucagon and insulin release. 5
PATIENT ASSESSMENT
The endocrine system affects most, if not all, body systems. Complaints most commonly associated with endocrine disorders include fatigue and weakness, polyuria, polydipsia, weight changes, and mental status changes. The focused assessment should target the specific presenting complaint.
When a critically ill patient presents to the ED, an endocrine etiology should be considered as part of the differential diagnosis. Care of the patient begins with assessment and stabilization of the airway, breathing, and circulation (ABCs) as well as a neurologic assessment. The neurologic assessment includes evaluation of possible causes of altered consciousness such as trauma, blood glucose abnormalities, stroke, or toxins. Identification of a stressor or precipitating event may be elicited through patient history, family interviews, laboratory findings, radiographic analysis, or other diagnostic procedures.
SELECTED ENDOCRINE EMERGENCIES
The emergency nurse will encounter patients with varied severity of endocrine dysfunction. Selected conditions covered in this chapter include those situations that may lead to hemodynamic instability or altered neurologic function. Awareness of the potential for endocrine system abnormalities in every critical patient is extremely important for the ED nurse.
Diabetic Emergencies
Diabetes mellitus is a chronic condition characterized by hyperglycemia and disturbances of carbohydrate, fat, and protein metabolism. Two major types of diabetes mellitus exist: type 1, or insulin-dependent diabetes, is due to an absolute insulin deficiency; type 2, non–insulin-dependent diabetes mellitus or adult-onset diabetes, is characterized by impaired glucose secretion, peripheral insulin resistance, and increased hepatic glucose production. Type 2 is the most common type, accounting for approximately 90% of all diabetes in North America; obesity is a major risk factor for the development of this endocrine disorder.
Diabetic Ketoacidosis
Diabetic ketoacidosis (DKA) develops when a patient experiences relative or absolute depletion of circulating insulin. This potentially life-threatening condition may occur in previously undiagnosed patients, leading to the initial diagnosis of diabetes (particularly type 1). Infection, illness, pregnancy, or situational stressors can lead to gluconeogenesis, the manufacture of glucose from noncarbon substrates by the body. This creates a relative insulin deficiency. In some patients, excess circulating glucose secondary to poor dietary management can overwhelm an already stressed system. Absolute deficiency can occur in patients who fail to follow their prescribed insulin regimen or experience a stressor such as an illness and fail to take adequate insulin. The latter example demonstrates why diabetic education is critical.
After prolonged insulin deficiency the patient can present with four acute problems: hyperglycemia, dehydration, electrolyte depletion, and metabolic acidosis (Figure 37-2). Stress causes release of counterregulatory hormones, which can lead to gluconeogenesis. Severe hyperglycemia increases serum osmolality and leads to osmotic diuresis, resulting in significant dehydration. Osmotic diuresis leads to urinary losses of water, sodium, potassium, magnesium, calcium, and phosphorus. The typical total body water loss in DKA is 6 L in adult patients.
![]() |
FIGURE 37-2 Pathophysiology of diabetic ketoacidosis. |
It is thought that the hyperosmolarity further impairs insulin secretion and promotes insulin resistance. Without insulin, the newly liberated glucose cannot be used, further increasing serum blood glucose levels, urine glucose concentrations, and osmotic diuresis. 3 Fats and muscle proteins are metabolized for energy. The ensuing lipolysis causes a buildup of fatty acids, which overcomes the body’s natural buffering system and leads to ketoacidosis.
DKA develops over a relatively short time, usually 2 to 3 days. The condition occurs most often in young adults and can result from infection, illness, stress, substance abuse, or failure to adhere to an insulin regimen. The patient describes a steady progression of symptoms, including polydipsia, polyuria, fatigue, and weakness. Patients with new-onset diabetes report recent weight loss. As ketoacidosis worsens, nausea, vomiting, decreased appetite, and abdominal cramping occur. The patient appears in moderate to severe distress with possible alteration in level of consciousness, confusion about recent events, or slow response to questions. Lethargy can progress to coma, and hyperthermia may be present.
Rapid, deep, Kussmaul respirations are present, and a fruity odor is usually noted on the breath. This acetone smell indicates worsening ketoacidosis. The cause of Kussmaul breathing is a respiratory compensation for a metabolic acidosis. Blood gas values from a patient with Kussmaul breathing will show a decreased PCO 2 level because of a forced increased respiration (blowing off the CO 2). The patient feels an involuntary urge to breathe rapidly and deeply. The skin is usually hot and dry, skin turgor is diminished, and mucous membranes are dry. Hypotension can result from severe dehydration. The most frequently seen cardiac rhythm is sinus tachycardia, but dysrhythmias related to electrolyte disturbances also occur. This is a grave situation in the pregnant woman because of deleterious effects on the fetus. Early identification and aggressive interventions are critical to minimize effects on the fetus and the mother.
Initial laboratory studies should be obtained early and fluid therapy started on arrival to the ED. A serum glucose level higher than 300 mg/dL and normal or elevated potassium levels are usually present. Creatinine and blood urea nitrogen (BUN) levels may be elevated as a result of dehydration. Serum osmolality is greater than 310 mOsm/kg, and serum acetone titrations are positive. Serum ketone testing based on the measurement of β-hydroxybutyrate (BOHB or β-OHB, the main ketoacid causing acidosis) is recommended for diagnosis and monitoring. Mean BOHB levels in DKA are about 7 mmol/L, but can range from 4 to 42 mmol/L. 3 Urinalysis results include elevated levels of ketones and glucose. Blood gas results typically demonstrate normal arterial oxygen pressure (PaO 2), respiratory alkalosis, and metabolic acidosis. Infection can precipitate DKA, so blood cultures and a urine culture should be obtained when infection is suspected. After cultures are obtained, antibiotic therapy should begin. Radiographs may also be required to determine the primary site of infection.
Management in the ED should be aggressive. Treatment focuses on correction of hyperglycemia, dehydration, electrolyte imbalances, and metabolic acidosis. Close monitoring with frequent reassessment is essential to prevent complications. Serum glucose and potassium levels should be monitored every 1 to 2 hours, and pH should be monitored every 2 to 4 hours. 3 Oxygen therapy should be initiated for any signs of hemodynamic compromise or altered mental status.
Intravenous fluid replacement should start immediately with 1 to 2 L of normal saline over the first 1 to 2 hours of treatment for adults. In children the initial fluid bolus is weight based (5 to 20 mL/kg, dependent on the child’s perfusion status); volume replacement is carefully titrated because of the high risk for cerebral edema in the pediatric population. The adult patient may require up to 8 to 10 L of fluid. Volume is gradually replaced after the initial fluid bolus because rapid infusion of a large volume increases the risk for development of cerebral edema. Close observation of intake and output is essential; placement of a urinary catheter ensures accurate output assessment. A continuous infusion of regular insulin is administered at 0.1 units/kg/hr to stop ketogenesis and achieve a steady decrease in serum glucose level of 50 to 75 mg/dL/hr; an initial intravenous (IV) bolus of 0.15 units/kg of regular insulin may be administered. The short duration of action for regular insulin allows better control of serum glucose levels. After serum glucose level reaches 250 to 300 mg/dL, fluids should be converted to 5% dextrose in normal saline (D 5NS) to provide fuel until the patient is able to eat. Resolution of a hyperglycemic emergency occurs when the serum glucose level is less than 200 mg/dL, serum bicarbonate level is greater than or equal to 18 mEq/L, and in DKA, the venous pH is greater than 7.3. 3
Fluid replacement dilutes serum potassium and promotes diuresis. In addition, total body hypokalemia is exacerbated by metabolic acidosis, so potassium replacement should begin after the initial liter of IV fluids is infused, even when initial values are normal. Potassium levels frequently drop precipitously in the first few hours after treatment has been initiated because potassium moves back to the intracellular space along with the insulin and existing glucose. Serum potassium levels must be repeated every 1 to 2 hours during initial management. Cardiac monitoring is essential because dysrhythmias can develop with significant hypokalemia.
Acidosis generally corrects with insulin therapy. Insulin allows the cells to use available glucose for energy, leading to decreased proteinolysis and lipolysis, and the ketoacidosis resolves. Insulin infusion should be continued until the pH or serum bicarbonate level has normalized; IV fluids should be converted to D 5NS once the serum glucose level reaches 250 to 300 mg/dL to prevent hypoglycemia. Acidosis in DKA is not routinely treated with sodium bicarbonate because sodium bicarbonate administration can cause rebound alkalosis, which can worsen hypokalemia and increases the risk for development of cerebral edema.
Controlling nausea and vomiting not only improves patient comfort but prevents worsening dehydration. The patient may require analgesia to relieve abdominal pain, headaches, or other somatic complaints. Providing a quiet, calm environment can improve patient comfort. Stress reduction plays an important part in patient recovery. Thorough explanation of treatment, medications, and plan of care can alleviate stress related to hospitalization.
Potential complications include hypoglycemia, hypokalemia, dysrhythmias, and cerebral edema. Monitor capillary/serum glucose levels, electrocardiogram (ECG), laboratory values, vital signs, intake and output, and neurologic status carefully. If the serum glucose level falls rapidly, the resulting fluid shift can lead to cerebral edema, which is associated with a higher mortality rate. Cerebral edema remains the leading cause of death for children presenting in DKA.
Hyperosmolar Hyperglycemic State
Hyperosmolar hyperglycemic state (HHS), also known as hyperosmolar hyperglycemic nonketotic coma (HHNC), is a life-threatening emergency characterized by marked elevation of blood glucose level, hyperosmolarity, and little or no ketosis. HHS threatens type 2 (non–insulin-dependent) diabetic patients with mortality rates as high as 10% to 50%, although true mortality data are difficult to interpret due to the high incidence of comorbidities. 19 Delayed diagnosis in undiagnosed type 2 diabetes, alcoholics, and dialysis patients place these groups at the greatest risk.
With the increase in the prevalence of type 2 diabetes and the aging population, this condition is likely to be encountered more frequently in the future. The precipitating causes are numerous, with underlying infections, acute myocardial infarction, and stroke being the most common. Other causes include certain medications, medication and treatment noncompliance, undiagnosed diabetes, substance abuse, and coexisting disease. Physical findings include those associated with profound dehydration and various neurologic symptoms such as coma.
Typically, patients presenting with HHS are over 50 years of age and have undiagnosed diabetes or type 2 diabetes managed by diet and/or oral medications. In addition, they frequently are on medications that aggravate the problem, such as diuretics that cause mild dehydration. 19 Type 2 diabetic patients over 50 years typically present with other contributing health problems, so it is necessary to search for other underlying illness. When stressed with illness, surgery, or injury, the body responds with increased glucose levels. Type 2 diabetic patients produce enough insulin to avoid ketoacidosis but not enough to prevent profound hyperglycemia.
Increased serum glucose levels act as osmotic diuretics, leading to severe dehydration. Patients are unable to replace lost fluids, so their condition progressively worsens. Lack of sufficient circulating insulin causes the body to metabolize fat and muscle tissues, which increases circulating fatty acid and amino acid levels. The resulting hepatic gluconeogenesis compounds existing hyperglycemia. Underlying renal disease or decreased intravascular volume will decrease the glomerular filtration rate and cause the glucose level to increase. The loss of more water than sodium leads to hyperosmolarity. Insulin is present, but it is not sufficient to reduce blood glucose levels, particularly in the presence of insulin resistance. 19Figure 37-3 illustrates the pathophysiology of HHS.
![]() |
FIGURE 37-3 Pathophysiology of hyperosmolar hyperglycemic nonketotic coma. |
Onset of symptoms in HHS is much more insidious than with DKA, developing over days or even weeks. Subtlety of symptoms may account for delay in seeking treatment. The patient may notice vague abdominal pain, decreased appetite, polydipsia, and polyuria. As the disease progresses, neurologic symptoms such as headaches, blurred vision, and confusion develop. Changes in level of consciousness, seizures, or coma also occur. Tachycardia, dysrhythmias, and hypotension may also be present. Respirations may be increased but do not have the fruity smell associated with DKA. It is the decrease in mental status that usually brings the patient to the hospital.
Laboratory analysis includes complete blood count (CBC), electrolytes, urinalysis, and arterial blood gases. Typical laboratory findings in HHS include blood glucose levels greater than 600 mg/dL (33.3 mmol/L), serum osmolality greater than 320 mOsm/kg, pH levels greater than 7.30, and mild or absent ketonemia. Elevated white blood cell (WBC) count is present with infection. Serum sodium and potassium levels may be normal or elevated depending on the level of dehydration.
The treatment of HHS involves vigorous IV rehydration, correction of electrolyte imbalances, administration of IV insulin, and diagnosis and management of underlying illness and coexisting conditions. Due to the altered level of consciousness, oxygen therapy should be initiated immediately. Rapid IV fluid replacement is initiated with isotonic crystalloid solution. Continual assessment and reassessment are the keys to preventing complications associated with fluid therapy. Most patients are older than 50 years, so circulatory overload is a marked risk with rapid fluid therapy. Urinary catheter placement assists with monitoring of intake and output. Intravenous potassium may be indicated to prevent hypokalemia from hemodilution and insulin therapy. Continuous cardiac monitoring is essential due to the risk for dysrhythmias related to the potassium deficits. Once fluid replacement has been initiated, regular insulin should be administered as a continuous infusion of 0.1 unit/kg/hr; an initial bolus of 0.15 unit/kg regular insulin may be administered.
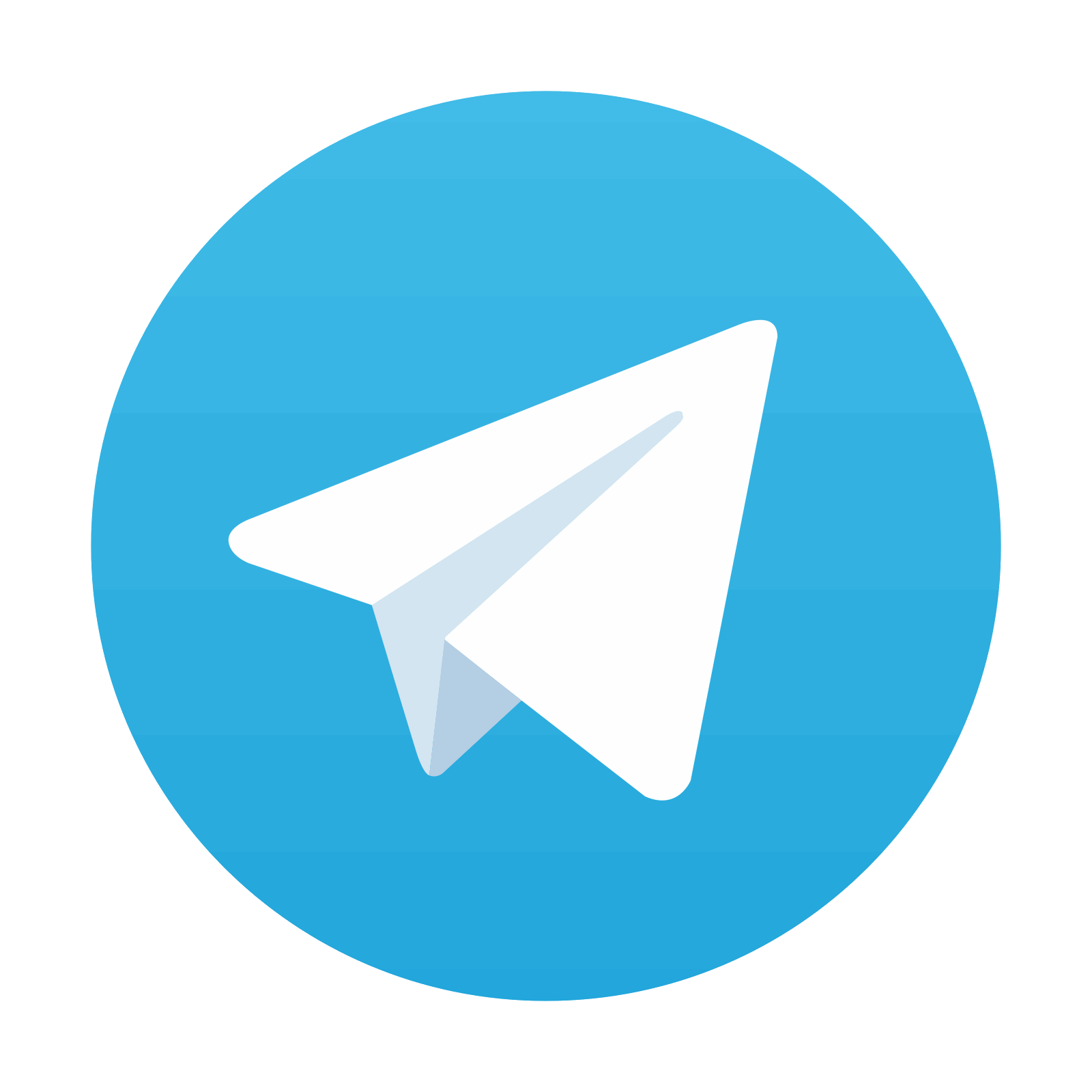
Stay updated, free articles. Join our Telegram channel

Full access? Get Clinical Tree
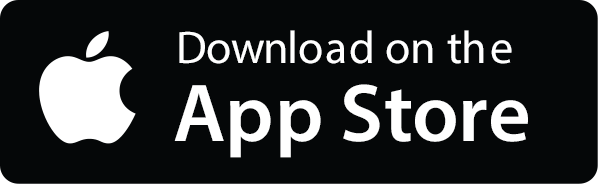
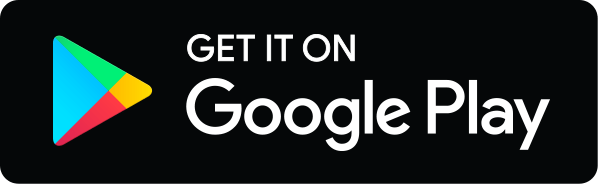
