- Normal defence against blood stream infection
- Factors that increase risk of blood stream infection
- Bacterial species that cause blood stream infection
- Symptoms of blood stream infection
- Defining sepsis, SIRS and septic shock
- Collection of blood for culture
- Interpretation of blood culture report
In this second of two chapters highlighting the work of the clinical microbiology laboratory, attention is focused on blood culture, a test used to confirm the presence of bacteria or fungi in blood (bacteraemia or fungaemia). Blood culture is useful in three broad clinical contexts. The first is among patients who are suspected of suffering sepsis (systemic disease caused by bacterial and, much more rarely, fungal infection). Secondly, there are several infectious diseases, whose pathogenesis involves spread of bacteria via the bloodstream. These diseases include endocarditis (infection of the valves of the heart), osteomyelitis (infection of bone) and infective arthritis (infection of joints). A positive blood culture provides supportive evidence for diagnosis of these conditions. Finally, the third group of patients who are likely to have their blood cultured are those with PUO (pyrexia of unknown origin). This is usually defined as a raised body temperature for more than ten days, with no immediate explanation. The clinical investigation of a patient with PUO routinely includes a blood culture in the search for an infective cause of pyrexia.
Normal physiology
Blood is normally sterile (contains no bacteria or other microbes). However, normal life is associated with the risk of bacteria coming into contact with circulating blood, and transient bacteraemia may occur from time to time without ill effect. For example, bacteria normally present in the mouth have access to the blood stream during dental surgery; transient bacteraemia invariably follows dental surgery. Even vigorous chewing has been shown to facilitate entry of bacteria, normally present in the mouth, to the blood stream. Likewise a ‘dirty’ cut allows environmental bacteria, and bacteria normally present on the skin, access to the blood stream. Small numbers of bacteria or fungi may enter the blood stream from an existing infection site (e.g. the urinary tract, the respiratory tract, a wound infection etc.). Despite scrupulous aseptic technique, any surgical procedure may be associated with passage of bacteria from sites (e.g. the skin and bowel), where bacteria are normally present in abundance, to the blood stream.
It is the innate and acquired immune defences of blood that maintain its sterility, and prevent transient bacteraemia – which inevitably occurs from time to time – developing to clinically significant bacteraemia, usually called blood stream infection or septicaemia.
Innate immune defences in blood
Innate immunity is the sum of the protective mechanisms against infection that we are born with. One of the principle functions of skin, for example, is to act as a physical barrier to invading organisms; in this way skin makes a major contribution to the body’s innate immune defence. The most significant innate immune defence against bacterial invasion in blood is neutrophils, phagocytic white blood cells. These cells engulf invading bacteria by the process of phagocytosis. Once phagocytosed, bacteria are killed by enzymes and highly reactive ‘free radical’ chemicals, produced within the neutrophil. The process of phagocytosis is greatly enhanced by the so-called complement proteins, which also make a significant contribution to innate immunity. Production of these blood proteins is activated by some species of invading bacteria. One of the complement proteins, known as C3b binds to the surface of bacteria. Bacteria that are coated with C3b are much more easily phagocytosed by neutrophils. Other complement proteins (C6, C7, C8 and C9) kill or damage some species of bacteria directly by insertion in bacterial membranes, and some (e.g. C5a) act by attracting neutrophils towards bacteria, thus enhancing phagocytosis.
Acquired immune defence in blood
We are not born with this form of immune defence, rather it is acquired as a result of exposure to invading organisms. It is very specific; meaning that exposure to one invading bacterial strain provides immunity (protection) only for that bacterial strain. (By comparison, innate immune mechanisms are non-specific: for example, skin protects against invasion from all bacterial species.)
Acquired immunity depends on the presence of antibodies in blood. Bacterial invasion results in production of specific antibodies (immunoglobulins) to bacteria by plasma cells; these cells are derived from a sub-group of the white blood cell population called B-lymphocytes. Antibody binds to the specific protein (antigen) present on bacterial surface that induced its production by plasma cells. This antibody binding of bacteria has two damaging effects for the bacteria: firstly it enhances neutrophil phagocytosis of bacteria and secondly it enhances the bactericidal effect of complement proteins. Many bacteria produce chemical toxins; specific antibodies bind to these toxins, effectively neutralising them. Finally, antibodies can activate production of the protective complement proteins by an alternative pathway to that induced by bacteria.
Thus by the complex synergistic action of both innate and acquired immune mechanisms, any bacteria present in blood are destroyed and blood remains essentially sterile.
Bacteraemia and fungaemia
Pre-disposing factors
If bacteria (or fungi) invade the bloodstream and the normal (innate and acquired) defences against invasion are overwhelmed, blood stream infection ensues.
Contributory factors include:
- Reduced host defences.
- Existing focus of infection.
- Virulence of invasive organism.
- Hospital procedures that facilitate entry of bacteria to the blood stream.
Reduced host defences
Any condition associated with reduced immune (either innate or acquired) defence against microbial invasion, predisposes to blood stream infection.
So, for example, major burns victims are predisposed to blood stream infection because as a result of their injury they lack the innate immunity provided by an intact covering of skin.
Those with AIDS, and those prescribed cytotoxic drugs, are particularly vulnerable to blood stream infection because of the reduced innate and acquired immunity associated with marked reduction in white blood cell numbers. Such patients are often so severely immunosuppressed that they are vulnerable to so called opportunistic bacteria and fungi (i.e. bacteria or fungi of such low virulence that they never pose a threat for those with a functioning immune system).
Any chronic debilitating illness (e.g. cancer, renal failure, heart failure etc.) or major trauma is associated with some degree of reduction in the normal immune response to infection. Premature babies have an under developed immune system and are particularly prone to infection during the first few months of life. The immune response to infection becomes less effective with advancing years; blood stream infections occur much more commonly among the elderly (> 65 years), than among younger adults. Finally diabetic patients are more at risk of some bacterial infections spreading to the bloodstream than non-diabetics.
Existing focus of infection
In most cases of blood stream infection there is a pre-existing infection at some site (called the focus of infection) in the body. Bacteria from this primary site invade the blood. If the organisms are not susceptible to the bactericidal (bacterial killing) action of blood, or the numbers of organisms are overwhelming, bacteria multiply within the blood stream. The most common foci of infection in patients with bacteraemia are the lower respiratory tract and urinary tract, but bacteria may enter blood from any site of infection and, if the conditions are ‘right’, multiply within the blood stream.
Virulence of invading organism
The vulnerability of bacteria to blood defences varies between species so that, for example, Gram positive bacteria are generally speaking resistant to the bactericidal properties of antibody and complement, although they are vulnerable to phagocytosis. This variation determines that invasions by some species of bacteria (highly virulent bacteria) are more likely to result in significant bacteraemia than others of low virulence. However if there is an established focus of infection in the body and/or the patient’s defences are sufficiently impaired, any bacterial species no matter what its virulence can cause blood stream infection. In fact, although only a few species of bacteria cause most cases, all species of pathogenic (disease causing) bacteria and even some normally non-pathogenic species can cause clinically significant bacteraemia if present in large enough numbers, and host (patient) immune defences are sufficiently debilitated.
Hospital procedures that facilitate entry of bacteria to the blood stream
Around 60% of all patients with blood stream infection have acquired the infection whilst in hospital1. Patients at greatest risk of hospital acquired (nosocomial) bacteraemia are those who are subjected to surgical procedures, intravenous or urinary catheterisation, cystoscopy and other operative invasive interventions. An infected intravenous catheter (most commonly a central line) is the focus of infection in around 20% of all patients suffering hospital acquired bacteraemia and most of those patients with hospital acquired bacteraemia whose focus of infection is the urinary tract have been catheterised, or have had their urinary tract investigated using a cystoscope. Surgery of areas that are normally heavily contaminated with bacteria (e.g. the mouth, the colon, genital area) carry the highest risk of post-operative bacteraemia.
Causative bacteria
Usually a single species of bacteria is the cause of blood stream infection, but in around 8% of cases more than one bacterial species is found in blood1. All pathogenic (disease causing) bacteria and, more rarely, some usually non-pathogenic (opportunistic) bacteria have been implicated as causative in particular cases of blood stream infection. There are however a few species of bacteria which account for the vast majority of cases.
The two most common causes are the Gram-negative bacterium Escherichia coli (E.coli) and the Gram-positive bacterium Staphylococcus aureus (S.aureus)1,2. E.coli is the causative organism in around 25% of blood stream infections and S.aureus is the cause in around 12%, so together these two bacterial species account for close to a little over a third of all cases.
E.coli is present as part of the normal bacterial flora of the gastrointestinal tract, and many cases of blood stream infection associated with this organism result from disease or trauma of the abdominal area and resulting blood invasion of bacteria from the gut. Abdominal surgery, for example, is associated with risk of E.coli bacteraemia.
E.coli is responsible for most cases of urinary tract infection so that the focus of infection in many cases of E.coli blood stream infection is the urinary tract, particularly the upper urinary tract.
In recent years the incidence of E.coli blood stream infection has been steadily increasing. In an effort to understand this increase, and hopefully devise the means to combat it, mandatory reporting of all cases of E.coli blood stream was introduced in 2011; the only other blood stream infection that requires mandatory reporting in the UK is that caused by S.aureus (mandatory reporting introduced over a decade ago).
S.aureus is present without ill effect in the nose of 20–30% of the population and the skin of 5–10%. It is however also a common pathogen of the skin, being responsible for superficial skin infections such as boils and carbuncles. Surgical and trauma induced wound infections are frequently caused by S.aureus and the organism is responsible for most (>80%) cases of bone infection (osteomyelitis) and joint infection (septic arthritis). The most common foci of infection in cases of S.aureus blood stream infections are infected wounds and infected intravenous catheters. In around 10% of cases caused by S.aureus, the particular strain is resistant to methicillin and related antibiotics – it is an MRSA (methicillin resistant Staphylococcus aureus) strain. UK government led initiatives3 over the past decade aimed at reducing the incidence of hospital acquired infection have been particularly successful in combating blood stream infections caused by MRSA. The annual rate of MRSA blood stream infections has fallen year on year from 7700 in 2003 to 1100 in 20114. As a percentage of total S.aureus blood stream infections, those due to MRSA has fallen during that period from 40% to just 10%.
In excess of 150 species of bacteria are the cause in the remaining two thirds of cases. The vast majority of these are rare but the following are relatively common causes of blood stream infection, together accounting for around another third of cases: Streptococcus pneumoniae, Streptococcus pyogenes, Staphylococcus epidermidis, Pseudomonas aeruginosa, enterococcal species, Klebsiella species and Proteus species.
Streptococcus pneumoniae
A Gram-positive cocci, which may be present in the mouth and nose of healthy people, but is the most significant bacterial cause of the common lower respiratory tract infection, pneumonia. It is the causative organism in around 2–5% of cases of bacteraemia; most of these are the result of spread of the organism to blood from infected respiratory tract, in patients suffering pneumococcal pneumonia.
Streptococcus pyogenes
A common pathogen of the upper respiratory tract: responsible for pharyngitis (‘strep throat’); skin infections (e.g. impetigo, cellulitis) and serious invasive tissue infection (necrotising fasciitis). Strep pyogenes infections are usually community-acquired.
Staphylococcus epidermidis
This is the most significant of a group of staphylococcal bacteria known collectively as cogulase negative staphylococci (CNS). In common with all other species in the group, Staph epidermidis is abundantly present on the skin and inside the nose of healthy individuals and this determines that it is a common contaminant of blood cultures. However it can also be a causative organism in cases of blood stream infection, most particularly among immunocompromised patients who have undergone some invasive procedure (e.g. intravenous catheterisation). Blood stream infection caused by this organism is usually hospital acquired.
Pseudomonas aeruginosa
A Gram-negative bacillus (rod shaped), this is a common environmental bacterium that thrives on moist surfaces and can be found contaminating hospital environment, surfaces, equipment etc. Most cases of blood stream infection caused by this organism are related to infected venous or urinary catheters, so is usually hospital acquired.
Enterococcal species
These are Gram-positive cocci (sphere shaped) normally present in the gastrointestinal tract. Clinically, the most significant species are Enterococcus faecium and Enterococcus faecalis, which can be the causative organism in cases of abdominal infections (e.g. peritonitis) and urinary tract infection. Spread from these sites of infection to blood can occur in severely debilitated patients.
Klebsiella and Proteus species
These are a relatively common cause of urinary tract infection and much more rarely, lower respiratory tract infection (pneumonia). The urinary tract or infected wounds is usually the focus of infection among those with bacteraemia associated with these organisms.
Causative fungi
Fungi are the cause in fewer than 2% of cases of blood stream infection3. Almost all of these occur in patients who are severely immunosuppressed. The causative organism in almost all cases of fungaemia is Candida albicans, a fungus that is present in low numbers on the skin of healthy individuals. It is the cause of common superficial skin and nail infections and vaginal candidosis, a superficial infection of the vagina.
Consequences of bacteraemia/fungaemia
The symptomatic and potentially life threatening consequences of bacteraemia and fungaemia are due in large part, not to the invading organism, but to dysregulation of the body’s normal physiological response to infection, which has already been very briefly outlined. The detail of the dysregulation is complex, only partially understood and the object of intensive current research5,6. It is manifest clinically as sepsis, which can quickly progress to severe sepsis, septic shock and death. An outline understanding of the clinical significance of blood stream infection depends crucially on defining the following terms:
- Systemic inflammatory response syndrome (SIRS).
- Sepsis.
- Severe sepsis.
- Septic shock.
Systemic inflammatory response syndrome (SIRS)
Inflammation is the normally protective response to any injury that ensures limitation and resolution of injury (healing). In health the inflammatory response is limited to the site of injury and is exquisitely controlled by an opposing anti-inflammatory process. SIRS is defined as an abnormal (dysregulated) inflammatory response that has effect in organs or sites removed from the site of injury. It is thus inappropriate and harmful. SIRS can be caused by any major insult to the body, for example, severe trauma and major surgery; burns; diseases like acute pancreatitis that are associated with extensive tissue damage; and infection. A diagnosis of SIRS is made if patients exhibit at least two of the following abnormal signs of systemic inflammation:
| < 36°C or > 38°C. |
| heart rate greater than 90 beats/minute. |
| respiratory rate > 20 breaths/minute or PaCO2 < 4.2kPa. |
| > 12.0 × 109/L or < 4.0 × 109/L. |
Sepsis
Sepsis is defined as infection in the presence of SIRS. Thus the finding of bacteraemia in a patient exhibiting two or more of the qualifying SIRS signs allows a diagnosis of sepsis. Since bacteraemia is a systemic infection, eliciting a blood-borne systemic inflammatory response, sepsis is usual in those with bacteraemia. However bacteraemia is not necessary for a diagnosis of sepsis; focal infection at any site can elicit SIRS, so that a diagnosis of sepsis can be made even if blood is sterile, so long as there is evidence of infection somewhere in the body in association with systemic inflammation. The term septicaemia (infection of blood) is often used as a synonym for sepsis, but since sepsis can occur in the absence of blood infection they are not synonyms. Experts now argue that because of this confusion, use of the term septicaemia should be discouraged in favour of the term blood stream infection.
Severe sepsis
The dysregulated response to infection that sepsis represents is associated with inappropriate release of a host of potent chemicals (cytokines) to blood from a range of cells involved in the immune process. Unchecked, pro-inflammatory cytokines cause damage to the endothelial cells that line the microvasculature making capillaries leaky with loss of fluid to the interstitial space, hypovolaemaia and fall in blood pressure (hypotension). Inappropriate inflammation and damaged endothelium activates the clotting cascade as well as platelets, and a pro-coagulant state ensues in which micro-thrombi (small blood clots) form inappropriately within capillaries. The damaging cascade continues as the reduced blood pressure, presence of micro-thrombi and endothelial damage combine to reduce blood flow through the microvasculature. This leaves tissues deficient of the oxygen required for cells to survive and ischaemic tissue damage progresses to organ dysfunction; it is this organ dysfunction that defines severe sepsis
Progression to severe sepsis occurs unpredictably in around a third of sepsis cases, but may be prevented by early recognition and prompt antibiotic therapy. It is defined as sepsis with evidence of organ dysfunction, hypotension or poor tissue perfusion. The damaging effects on the microvasculature are widespread so any or all organ systems can be affected.
Septic shock
The most severe presentation of sepsis is septic shock, characterised by acute circulatory failure and persistent hypotension (systolic pressure <90 mmHg), despite adequate fluid resuscitation. Reduced tissue perfusion can lead to multiple organ failure and death.
Sepsis, severe sepsis and septic shock are not separate conditions but rather represent a continuum of severity of the same condition, namely an abnormal systemic response to microbial (usually bacterial) infection. Patients with blood stream infection may present at any stage. Severity is reflected in mortality rates: sepsis is associated with around 25% mortality, severe sepsis with 40% mortality and septic shock with 60% mortality7. Sepsis and its sequalae remains the most common cause of death among patients being cared for in intensive care units, and currently accounts for an estimated 37 000 deaths in the UK every year.
Following the collaborative effort of critical care expertise from around the world, the now influential Surviving Sepsis Campaign (http://www.survingsepsis.org) was launched in 2004. The campaign has allowed preparation of internationally agreed clinical guidelines8 aimed at significantly reducing sepsis related mortality. The so called ‘care bundles’ (which include the use of blood culture and other laboratory tests) promoted by these guidelines are now being applied by nursing9 and medical staff in emergency and intensive care units at hospitals around the world.
Summary of the general symptomatic effects of bacteraemia
Infection caused by particular bacterial species may be associated with specific symptoms. The most common general symptoms are:
- Fever (body temperature >38 °C).
- Rigors (shivering chills).
- Tachycardia (heart rate >95 beats/minute).
- Increased respiratory rate.
- Alteration of mental state (confusion, apprehension).
- Hypotension (reduced blood pressure).
Signs and symptoms associated with organ dysfunction in severe sepsis include:
- Jaundice (liver).
- Increased tendency to bleed (coagulation defects).
- Reduced urine output (kidney).
- Respiratory distress, breathlessness – reduced PaO2 (respiratory system).
Principles of microbiological examination of blood
The primary objective of the laboratory is to determine if the patient’s blood contains bacteria (or fungi). It is not possible to confirm or exclude the presence of bacteria in blood by merely examining a sample under the microscope: there simply are not sufficient numbers present. Instead bacteria must first be grown (cultured) in a liquid (called the culture medium) that contains the nutrients necessary for bacteria to multiply. The culture medium containing the blood sample is incubated at 37°C the optimum temperature for bacterial growth, until there is evidence of bacterial growth. This usually takes 6–18 hours, but may take days for particularly slow growing species. In practice, if there is no evidence of bacterial growth after three days of incubation it is highly unlikely that the culture (and therefore the blood sample added to the culture medium) contains any bacteria. However the culture may continue to be monitored for longer to allow for the possibility that rare, slow growing species were present in the blood sample.
As soon as there is evidence of bacterial growth, a sample of the culture, now rich in bacteria, is stained and examined under the microscope. This provides the first evidence of the identity of the species of bacteria present (e.g. whether Gram positive or Gram negative, cocci or baccilli etc.). More precise identification may require that the liquid culture be further grown (sub-cultured) on a solid culture medium in a petri dish. This allows the growth of visible, pure colonies of bacteria, each colony being the product of multiplication of a single bacterium. A sample of the visible colony is then subjected to a range of chemical tests that finally determine the identity of the bacteria it contains.
Having isolated and identified the bacteria present in the culture, the final step is sensitivity testing. This involves testing the bacteria isolated in culture for reaction with a range of antibiotics, to establish which antibiotic is likely to be most effective in combating this particular infection.
Bacteraemia and fungaemia are potentially life-threatening conditions which demand immediate antibiotic treatment. It is often not practicable therefore to wait for the results of laboratory tests before starting treatment, and initial antibiotic therapy must be based on the clinical history, which provides important clues as to the likely nature of the invading bacterial species. However when the results of laboratory tests, particularly sensitivity testing, become available (usually within a day or two) antibiotic therapy can be altered if necessary.
Sample collection
Objective
To introduce a sample of patient’s blood into culture bottles without any bacterial contamination (e.g. from environment, operators or patient’s skin etc.).
Timing of sampling
Blood for culture should be sampled before administration of antibiotic therapy as antibiotics may delay or prevent bacterial growth, causing falsely negative results. For patients with intermittent fever, blood should ideally be taken while temperature is rising, or as soon after the spike of temperature as is possible, when the bacteria are present in blood at highest concentration. Most laboratories recommend taking a second or third sample, not less than one hour after the first to increase the chances of recovering bacteria and to distinguish true bacteraemia (which would be present in all culture sets) from bacterial contamination.
Blood culture bottles
Blood must be collected into specially designated blood culture bottles. There are several commercially available blood culture systems, but they all contain a sterile liquid mixture of nutrients (called the culture medium) necessary for bacterial growth. Most laboratories supply two blood culture bottles per blood culture set. The first has oxygen in the space above the culture medium to allow growth of those species of bacteria that require oxygen. The second bottle has a mixture of gases without oxygen. This bottle is required for culture of anaerobic bacteria (i.e. bacteria which only grow best in an oxygen-free environment). A sample of blood must be introduced into both bottles.
Volume of blood required
In a patient with bacteraemia there may be as few as one bacterium per millilitre of blood, so that a falsely negative result can occur if insufficient blood is introduced into the culture bottle. Paradoxically, a false negative result can also occur if too much blood is introduced. This is because blood continues to have bactericidal effect in culture. This effect is diluted out in the liquid culture medium. A compromise must be sought between too small a volume of blood, which might well contain insufficient bacteria and too large a volume, which would remove the dilution effect of the culture medium on bactericidal property of blood. An approximate 1:10 dilution of blood in culture medium is optimal but the actual volume required (usually 5–10 ml) depends on the blood culture system being used. It is vital that no less than the local laboratory recommended minimum volume of blood be sampled for each culture bottle.
Technique
Aseptic technique is essential throughout to ensure that no bacterial contamination of the culture occurs. If successful, only bacteria present in the patient’s blood will be transferred to the culture bottle.
- Blood should be sampled from a peripheral vein, not via an indwelling catheter, which might itself be contaminated with bacteria.
- With sterile gloved hands, the venipuncture site must be cleansed with 2% tincture of iodine, or some other suitable disinfectant. The iodine should be removed after a minute or two with 70% alcohol, ensuring that the site is dry. The top of both blood culture bottles, through which the sample is introduced, must be similarly disinfected.
- Taking care not to touch the venipuncture site, blood is collected using sterile syringe and needle.
- Blood is inoculated into the blood culture bottle via the rubber septum in the blood culture top. Never remove the top of a blood culture bottle. This would expose the culture to environmental bacteria.
- If blood is being collected for other tests, always inoculate blood culture bottles first, to prevent bacteria present on other specimen bottles being transferred to the culture.
- Blood culture bottles must be carefully labelled with patient details and sent along with the appropriate request card to the laboratory without delay. If blood is collected out of normal laboratory hours, blood cultures must be placed in a specially designated 37°C incubator to facilitate bacterial growth.
It is important to record on the request card outline clinical details and any antibiotic therapy if given before blood sampling.
Blood culture reports
Interim reports of progress in examination of a blood culture are issued daily. A final report will include the identity of any bacteria recovered from the culture, along with a report of the sensitivity or resistance of that particular strain to a range of antibiotics.
Results of blood culture fall into one of three main groups:
- Blood culture negative – no bacterial growth.
- Blood culture positive – pure growth.
- Blood culture positive – mixed bacterial growth.
Blood culture negative – no bacterial growth
This is a normal result, that is one which would be obtained from a person whose blood was sterile (contained no bacteria). Before a negative culture result is interpreted in this way, it is important to consider the possibility that the result is falsely negative, that is the patient has bacteraemia but the test has failed to detect it. Causes of false negative results include:
- Insufficient blood added to culture bottle.
- Antibiotic therapy administered before blood sampled.
- Incubation period insufficient for growth of rare, slow growing organisms.
Blood culture positive – pure growth
This means that a pure growth of a single identified species of bacteria (e.g. E.coli, Strep pneumoniae, Staph aureus etc.) was isolated from the culture. This is the result that would be expected from a patient with blood stream infection. However, in around 10–20% of positive blood cultures, the bacteria isolated and identified is derived not from the patient’s blood but is present as a result of bacterial contamination of the culture due most often to poor aseptic technique at the time of sample collection. Since all species of bacteria have been implicated in bacteraemia, and as a cause of sepsis at one time or another, it is sometimes difficult to decide whether a positive blood culture is due to contamination (false positive) or reflects bacteraemia and possible sepsis (true positive).
To illustrate this, suppose a blood culture yields a pure growth of the organism Staph epidermidis. This organism, which is normally present in abundance on the skin of us all, could be transferred from the skin of patient or staff to the blood culture during the process of blood collection. In fact it is one of the most common organisms to contaminate blood cultures. However, Staph epidermidis is also a quite common cause of sepsis among debilitated patients whose focus of infection is an infected catheter. It is also the most significant cause of endocarditis among patients who have received heart surgery. The finding may reflect contamination, but in some circumstances can be of clinical significance.
It is the bacteria, like Staph epidermidis, that are part of the normal resident flora of skin that usually contaminate blood cultures. A pure growth of any organism in blood culture is more likely to be due to bacteraemia than contamination, if:
- The same organism has been isolated from the same patient at some other infected site.
- The same organism is isolated from repeated blood cultures.
Blood culture positive – mixed bacterial growth
This result indicates that more than one species of bacteria was isolated from the blood culture. It is rare for blood to be infected by more than one species of bacteria, although it may occur. A mixed growth of bacteria suggests that the culture was contaminated, particularly if the bacterial species isolated are common contaminants. Interpretation may be difficult and frequently requires the expert help of a clinical microbiologist.
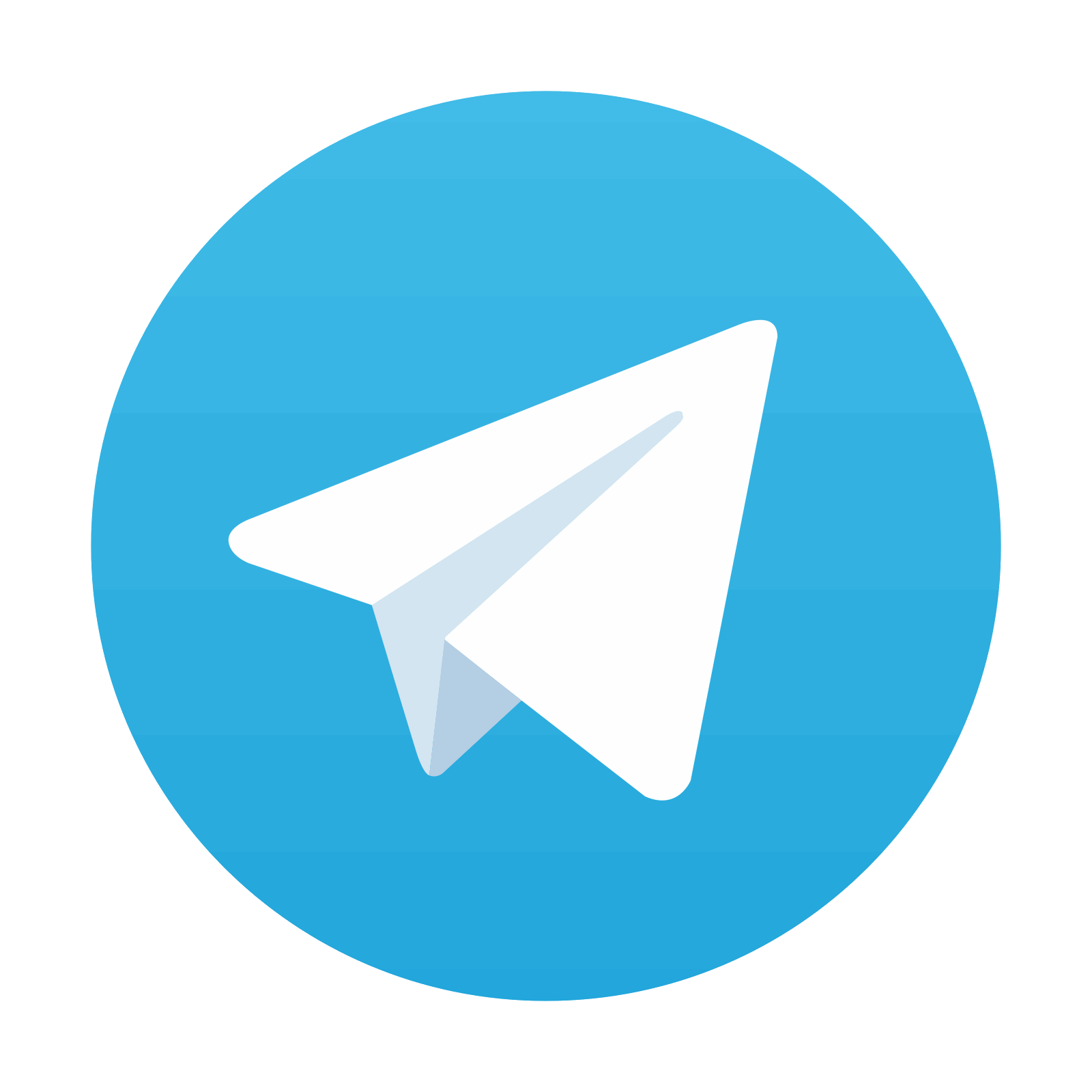
Stay updated, free articles. Join our Telegram channel

Full access? Get Clinical Tree
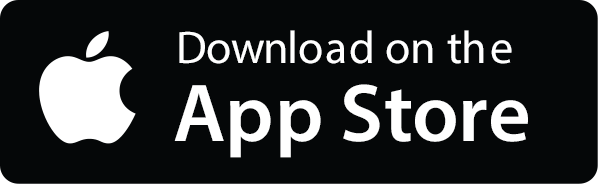
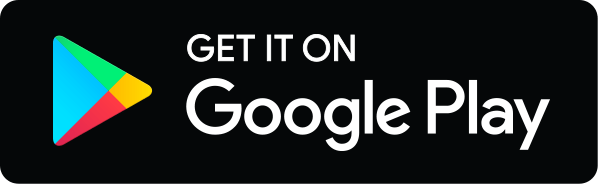