CHAPTER 21. Head Trauma
Beth Broering
Traumatic brain injury (TBI), a leading cause of death and permanent disability, is a major public health problem both in the United States and internationally. Over 2 million persons in the United States sustain a brain injury each year, more than 8 times the number of people diagnosed with breast cancer and 34 times the number of people diagnosed with human immunodeficiency virus/acquired immunodeficiency syndrome. 10.18. and 22. There are an estimated 50,000 deaths, over 200,000 hospitalizations, and more than 1 million emergency department (ED) visits. 18 TBI has resulted in more than 5 million persons in the United States living with permanent disabilities, many requiring lifelong assistance with activities of daily living. 18 According to the Centers for Disease Control and Prevention (CDC), the estimated direct and indirect costs of TBI were more than 60 billion dollars in 2000, with the average lifetime cost per survivor of TBI requiring hospitalization being $111,578 and the average cost per fatality being $454,717. 10.18. and 30.
Mechanisms of injury include blunt, penetrating, and blast forces that disrupt the vascular and neuronal structures inside the cranial vault, leading to a complex cascade of cellular and biochemical processes. A small percentage of patients with severe TBI will have concomitant fracture of the cervical spine. Falls are a common mechanism of injury in all ages but particularly in the pediatric population and those over 65 years of age. Persons of all ages sustain head injuries from motor vehicle crashes; however, those 15 to 24 years of age are at greatest risk. Other motorized vehicles (e.g., all-terrain vehicles, motorcycles, dirt bikes) have become an increasing cause of TBI in children and young adults. 5.9. and 21. Along with mechanisms, the forces of energy are important to understand. Brain injuries can result from acceleration, deceleration, rotational, or deformation forces. Table 21-1 provides an overview of the common energy forces associated with TBI.
Type of Force | Description | Result |
---|---|---|
Acceleration forces | When the head is struck by a moving object | Skull fractures Contusions Hematomas |
Deceleration forces | When the head is moving and strikes a stationary object (e.g., head hits the steering wheel of a car, ejected occupant hits head on the ground) | Skull fractures Contusions Coup-contrecoup injuries Hematomas |
Acceleration-deceleration forces | Combination of injuries due to rapid changes in the velocity of the brain | Coup-contrecoup injuries Diffuse axonal injuries Hematomas |
Rotational forces | Side-to-side and twisting movement of brain tissue | Diffuse axonal injuries |
Deformation forces | Direct blows or compression of the skull with resultant change in shape of the skull | Severity and extent of injury often determined by the velocity of blow or the length of compression |
Penetrating injuries occur most commonly from firearms but can also result from any sharp object that penetrates the scalp and skull. The extent of damage to brain tissue is determined by the point of entry, depth and angle of entry, and force of entry. Although all types of penetrating injuries are potentially lethal, gunshot wounds have the highest associated mortality rate. Blast injuries, the most common cause of TBI for military troops deployed to war zones, can occur in any type of explosion. Blast injury is often a combination of both blunt and penetrating forces. The impact of the blast waves moving through the victim’s body causes shearing of neuronal structures, while flying debris might produce penetrating injuries.
It is important for the emergency nurse to have an understanding of the mechanisms of injury and forces involved to ensure appropriate management and minimize the potential for secondary brain injury, complications, and missed injuries.
This chapter begins with a brief review of anatomy and physiology. It also provides an overview of assessment techniques focusing primarily on adult patients with TBI. Current management options for TBI are also discussed. For a more complete discussion on pediatric injuries, refer to Chapter 28.
ANATOMY AND PHYSIOLOGY
Anatomy
The hair, scalp, skull, meninges, and cerebrospinal fluid (CSF) protect the brain from injury (Figure 21-1). Five layers of tissue form the scalp: skin, subcutaneous tissue, galea aponeurotica, ligaments, and periosteum. The cranium, composed of the frontal, parietal, temporal, and occipital bones, joins with the facial bones to form the cranial vault, a rigid, nonexpandable cavity that can hold a volume of approximately 1700 mL. Bones of the cranium consist of three layers (Figure 21-2). The outer and inner tables are composed of hard cortical or compact bone. The diploë or middle layer is made up of soft cancellous bone. The structure of the cranial bones provides significant protection to the brain parenchyma. The skull is divided into the supratentorial and the infratentorial space. The cerebral hemispheres and the diencephalon are contained in the supratentorial space. The infratentorial space contains the cerebellum and the brainstem. Other bony structures of importance are depressions at the base of the skull called the anterior, middle, and posterior fossae. The frontal lobe is located in the anterior fossa. The deeper middle fossa contains the parietal, temporal, and occipital lobes. The posterior fossa is the largest and deepest and supports the brainstem and cerebellum.
![]() |
FIGURE 21-1 Brain structures. (From Thompson JM, McFarland G, Hirsch J et al: Mosby’s clinical nursing, ed 5, St. Louis, 2002, Mosby.) |
![]() |
FIGURE 21-2 The three layers of “skull bone”: outer layer of compact bone surrounding cancellous bone. Note the fine structure of compact and cancellous bone. (From Thibodeau GA, Patton KT: Anatomy and physiology, ed 6, St. Louis, 2007, Mosby.) |
Three layers of meninges surround the brain and provide additional protection. The outermost meninx is the dura mater (meaning “tough mother”), which consists of two layers of tough fibrous tissue. The inner layer of the dura mater produces prominent folds that subdivide the interior of the cranial cavity. The largest of these folds forms the falx cerebri, which separates the brain into the right and left cerebral hemispheres. The next-largest fold is the tentorium cerebelli, which divides the posterior cranial fossa into the superior (supratentorial) and inferior (infratentorial) compartments. Potential spaces located above the dura mater (epidural) and below the dura mater (subdural) are at risk for hematoma formation because the middle meningeal artery lies in the epidural space, and veins are located within the subdural space. The middle meningeal layer is the arachnoid (spiderlike) mater, a fine, elastic layer. Below the arachnoid mater, the subarachnoid space is a relatively large space that is normally filled with CSF and contains arachnoid villi, fingerlike projections that form channels for CSF absorption. Adhering to the surface of the brain is the pia mater (meaning “tender mother”).
The cerebrum consists of two hemispheres separated by a longitudinal fissure. Each lobe of the cerebrum is responsible for specific functions. The frontal lobe coordinates voluntary motor movements and controls judgment, affect, and personality. Hearing, behavior, emotions, and dominant-hemisphere speech are controlled by the temporal lobe. Sensory interpretation occurs in the parietal lobe, whereas the occipital lobe is responsible for vision.
The cerebral hemispheres are connected with the midbrain by the diencephalon. The thalamus, hypothalamus, subthalamus, and epithalamus are located within the diencephalon (Figure 21-3). The hypothalamus has numerous key roles in hormonal regulation and metabolic functions, including temperature regulation; release of hormones from the pituitary gland and adrenal cortex; emotional behaviors such as fear, rage, and pleasure; and activation of the sympathetic and parasympathetic functions of the autonomic nervous system.
![]() |
FIGURE 21-3 Divisions of the brain. A midsagittal section of the brain reveals features of its major divisions. (From Thibodeau GA, Patton KT: Anatomy and physiology, ed 6, St. Louis, 2007, Mosby.) |
The cerebellum is located in the posterior fossa adjacent to the brainstem and separated from the cerebrum by the tentorium cerebelli. Primary functions of the cerebellum are integration of motor function, maintenance of equilibrium, and maintenance of muscle tone.
The brainstem consists of the midbrain, pons, and medulla (see Figure 21-3). Although each structure has important pathway functions, the medulla contains the cardiac, respiratory, and vasomotor centers. The reticular formation, also located in the brainstem, is the central component of the reticular activating system and is responsible for arousal, the lowest level of consciousness, which is interpreted as wakefulness. Along with the primary cardiorespiratory centers, the brainstem contains many ascending and descending pathways that carry impulses between the spinal cord and the brain. In addition, all cranial nerves (CNs) with the exception of CN I and CN II originate in the brainstem. Table 21-2 describes the function of each CN.
Cranial Nerve | Function | Physiologic Effects |
---|---|---|
I. Olfactory | Sensory | Smell |
II. Optic | Sensory | Vision |
III. Oculomotor | Motor | Extraocular movement of eyes, raises eyelid, constricts pupils |
IV. Trochlear | Motor | Allows eye to move down and inward |
V. Trigeminal | Motor and sensory | Facial sensation, mastication, and corneal reflex |
VI. Abducens | Motor | Allows eye to move outward |
VII. Facial | Motor and sensory | Movement of facial muscles, closes eyes, secretes saliva and tears |
VIII. Vestibulocochlear | Sensory | Hearing and equilibrium |
IX. Glossopharyngeal | Motor and sensory | Gag reflex, swallowing, and phonation |
X. Vagus | Motor and sensory | Voluntary muscles for swallowing, involuntary to visceral muscles (heart, lungs) |
XI. Spinal accessory | Motor | Turns head, shrugs shoulders |
XII. Hypoglossal | Motor | Tongue movement for swallowing |
The anatomic structure of the capillaries of the brain, the tight junctions between endothelial cells, and the surrounding neuroglia form the blood-brain barrier. The blood-brain barrier acts as a protective mechanism restricting the free movement of substances from the blood vessels into the interstitial spaces and CSF. The blood-brain barrier, although mainly protective in nature, can hinder the effectiveness of some drugs. In brain injury, the breakdown of the blood-brain barrier may potentiate cerebral edema.
Physiology
In the adult patient the skull is a closed box containing three volumes: the brain (80%), CSF (10%), and blood (10%). Intracranial pressure (ICP) is a dynamic state that reflects the pressure in the supratentorial space as exerted by the total of the three volumes, which under normal conditions is maintained in constant balance through multiple homeostatic mechanisms. Normal ICP is less than 10 mm Hg with an upper limit of approximately 15 mm Hg. If one or more of the volumes of the cranial contents increases, ICP will rise and, if not immediately corrected, will compromise cerebral blood flow. The Monro-Kellie hypothesis describes the concept of reciprocal changes in volume as a means of compensation and maintaining ICP. As one of the volumes increases, there must be reciprocal decreases in the other two volumes or ICP will rise. CSF is initially displaced out of the cranial compartment into the spinal subarachnoid space, and production of CSF is reduced. Once CSF is maximally displaced, there is vasoconstriction and compression of the cerebral venous system. Sustained ICP greater than 20 mm Hg represents intracranial hypertension. If ICP continues to rise, arterial blood flow is compromised. These compensatory mechanisms have a finite ability to reduce volume and maintain ICP. As the intracranial volume increases beyond the compensatory threshold, there are sharp increases in ICP (Figure 21-4). Failure to reduce ICP may cause ischemia and necrosis of brain tissue.
![]() |
FIGURE 21-4 Intracranial volume-pressure curve. ICP, Intracranial pressure. (Modified from Lewis SM, Heitkemper MM, Dirksen RF, editors. Medical-surgical nursing: assessment and management of clinical problems, ed 7, St. Louis, 2007, Mosby.) |
The brain requires a constant supply of oxygen and nutrients, primarily glucose, to maintain function. It receives 15% of the cardiac output and consumes approximately 20% of the body’s oxygen supply. Cerebral blood flow is maintained through highly sensitive and complex mechanisms of autoregulation. Cerebral autoregulation is the ability of the brain to maintain a constant blood flow over a wide range of metabolic demands and systemic mean arterial pressures (normally 50 to 150 mm Hg). 3.8.9.23. and 26. This is accomplished through vasoconstriction or vasodilation of the cerebral blood vessels. For example, if metabolic demands rise, the cerebral vessels will vasodilate to increase cerebral blood flow, oxygen, and glucose delivery. Cerebral blood flow also remains relatively constant with changes in systemic pressure. The cerebral vessels vasoconstrict when systemic pressure is high and vasodilate as systemic pressures begin to fall. Cerebral autoregulation can be impaired or lost, either locally or globally, after brain injury. When cerebral autoregulation is disrupted, cerebral blood flow becomes dependent on the systemic blood pressure.
Cerebral perfusion pressure (CPP) is the pressure gradient across the brain or the pressure difference between the arterial blood entering the brain and the venous blood exiting. Adequate delivery of oxygen and nutrients requires adequate CPP (i.e., CPP ≥50 mm Hg). 2.6.13. and 23. CPP plays an important role in regulating cerebral blood flow. As CPP falls, the cerebral vessels will vasodilate to maintain cerebral blood flow. If CPP drops too low, the cerebral vessels collapse, and cerebral blood flow will actually fall, resulting in ischemia and neuronal cell death. CPP is calculated by subtracting the ICP from the systemic mean arterial pressure (MAP) (Box 21-1).
Box 21-1
C erebral P erfusion P ressure
MAP − ICP = CPP
Example: Mean arterial pressure = 90 mm Hg
Intracranial pressure = 15 mm Hg
90 mm Hg − 15 mm Hg = 75 mm Hg
CPP, Cerebral perfusion pressure; ICP, intracranial pressure; MAP, mean arterial pressure.
PATIENT ASSESSMENT
After ensuring adequate control of airway, breathing, and circulation (ABCs), the emergency nurse should perform a neurologic assessment. The goals of the neurologic assessment in brain-injured patients include detection of life-threatening injuries and establishing a baseline assessment that can be used as a comparison in subsequent examinations. A complete neurologic assessment, including mental status, level of consciousness or Glasgow Coma Scale (GCS) score, pupillary size and reactivity, CN assessment, reflexes, and motor symmetry and strength, can be performed in the awake and hemodynamically stable patient. In a patient with hemodynamic instability or with comorbid conditions that prevent a complete neurologic examination, the patient’s neurologic status should be described in as much detail as possible. A subtle change in level of consciousness is often the earliest indication of deterioration in the head-injured patient. Box 21-2 describes the early and late signs and symptoms of increased ICP.
Box 21-2
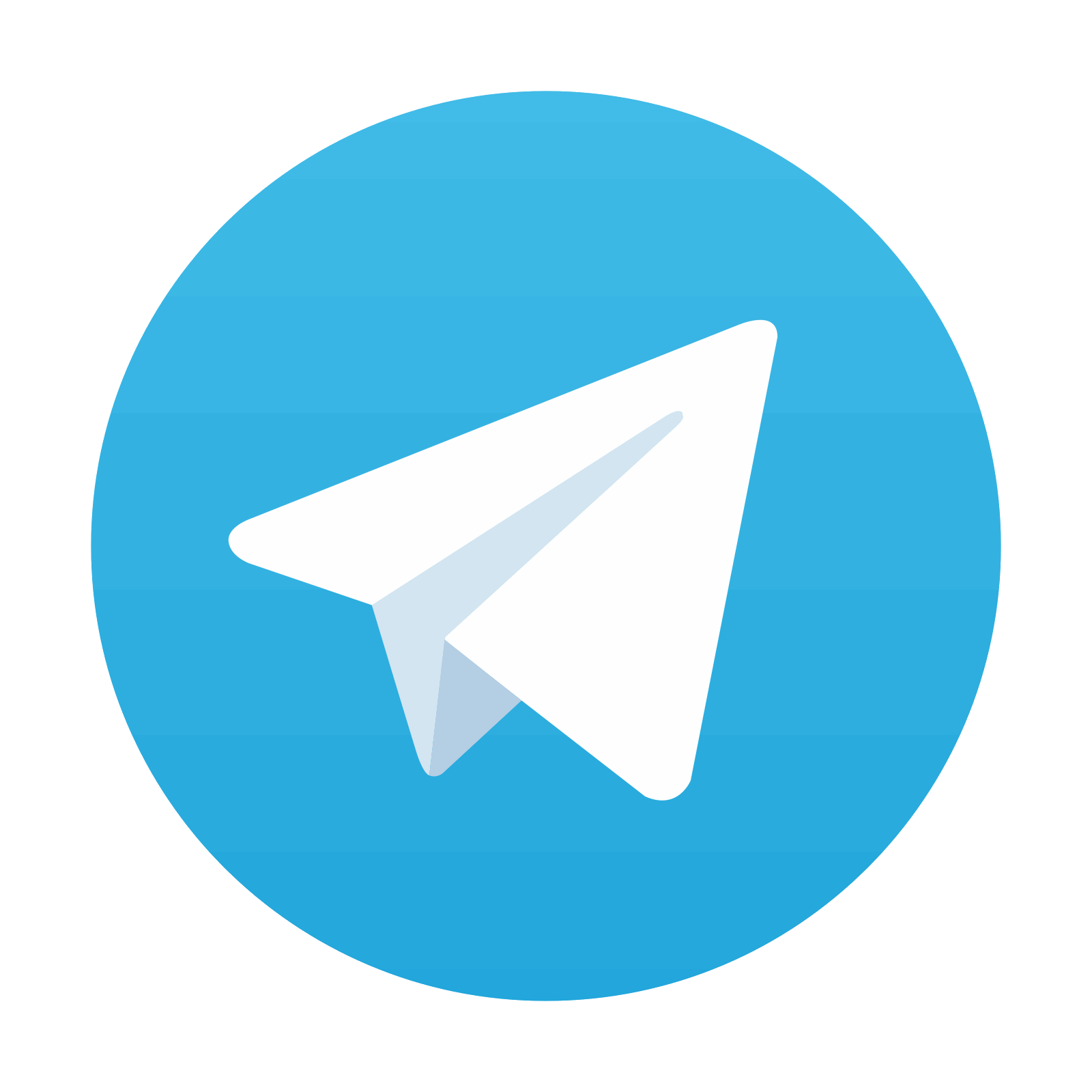
S igns and S ymptoms of I ncreased I ntracranial P ressure
EARLY
Level of conscious deteriorates: Patient may become, restless, more confused, agitated or combative
Headache
Nausea/vomiting
Slowed or slurred speech
Blurred vision or diplopia
Pupillary changes: Delayed/sluggish reactivity to light, pupil becomes ovoid, unilateral change in pupil size or shape
Decreased strength and sensation
LATE
Progressive decline in level of consciousness to coma
Projectile vomiting (without nausea)
Speech significantly impaired, may only groan
Impaired brainstem reflexes (corneal, gag)
Motor posturing
Unilateral or bilateral pupil that enlarges and becomes fixed
Irregular respirations
Cushing’s response
Cardiac dysrhythmias
Abnormal reflexes (Babinski’s)
Table 21-3 lists the components of the GCS, an objective, and universally accepted measure of a patient’s neurologic status. 27 Assessment of level of consciousness should be directed toward acquiring the highest-level or best response with the least stimulus. Completing the GCS allows assignment of numeric values to clinical changes. Interpretation of the GCS must be correlated with other clinical assessment findings. Other physiologic conditions such as hypotension, hypoxia, alcohol intoxication, or substance abuse may falsely lower the initial GCS. Eye and facial trauma may make assessment of eye opening inaccurate or difficult. Motor response may be difficult to assess in patients with spinal cord injuries. In addition, the emergency nurse must also consider the accuracy of response in the non–English-speaking patient. However, in the acute resuscitation a GCS score of 8 or less represents coma, and the nurse must assume the patient has sustained a severe head injury until further clinical and diagnostic studies can be completed.
CNS, Central nervous system. | ||
Response | Score | Significance |
---|---|---|
EYE OPENING | ||
Spontaneously | 4 | Reticular activating system is intact; patient may not be aware |
To verbal command | 3 | Opens eyes when told to do so |
To pain | 2 | Opens eyes in response to pain |
None | 1 | Does not open eyes to any stimuli |
VERBAL STIMULI | ||
Oriented, converses | 5 | Relatively intact CNS, aware of self and environment |
Disoriented, converses | 4 | Well articulated, organized, but disoriented |
Inappropriate words | 3 | Random, exclamatory words |
Incomprehensible | 2 | Moaning, no recognizable words |
No response | 1 | No response or intubated |
MOTOR RESPONSE | ||
Obeys verbal commands | 6 | Readily moves limbs when told to |
Localizes to painful stimuli | 5 | Moves limb in an effort to remove painful stimuli |
Withdrawal | 4 | Pulls away from pain in flexion |
Abnormal flexion | 3 | Decorticate rigidity |
Extension | 2 | Decerebrate rigidity |
No response | 1 | Hypotonia, flaccid: suggests loss of medullary function or concomitant spinal cord injury |
Normal pupillary response to direct light examination is constriction. Consensual reaction (constriction of the opposite pupil) should occur with direct light examination. Anisocoria or unequal pupils are a normal finding in 15% to 17% of the population, so assessment of reactivity in the dilated pupil is critical. Sluggish pupillary response may be the first indication of increasing cerebral edema and rising ICP. An oval pupil is also commonly seen in patients with increasing ICP. 2 With aggressive intervention the oval pupil will often return to normal size, shape, and reactivity as ICP is reduced. If ICP cannot be controlled, the pupil will become dilated and nonreactive. CN III exits the brainstem and lies at the junction of the midbrain and the tentorial notch. Any increase in downward pressure at the tentorial notch compresses the third CN, resulting in unilateral pupil dilation (Figure 21-5). Bilateral fixed and dilated pupils are indicative of impending transtentorial herniation.
![]() |
FIGURE 21-5 Uncal herniation with oculomotor nerve compression. (From Barker E: Neuroscience nursing: a spectrum of care, ed 3, St. Louis, 2008, Mosby.) |
The oculomotor (CN III), trochlear (CN IV), and abducens (CN VI) nerves control extraocular eye movements. In the conscious patient, extraocular movements should be assessed. Conjugate gaze is movement of both eyes simultaneously in the same direction. This indicates the brainstem and cerebral cortex are functioning. Disconjugate gaze is when one eye is deviated from the normal midposition with the patient at rest. Ask the patient to follow a finger through the six directions of gaze. If any of the three CNs are injured, there will be paralysis or paresis of the extraocular muscles, leading to a disconjugate gaze. Ptosis (drooping eyelid) may also be observed with injury to the oculomotor nerve. Patients may also complain of diplopia as the eyes move through the different positions. Injuries can be unilateral or bilateral; therefore it is important to assess each eye separately and observe for consensual and/or conjugate response.
With severe brain injury it is important to evaluate the integrity of brainstem function. The oculocephalic (doll’s eye) reflex tests the integrity of pontine centers. 2. and 6. A doll’s eye examination is performed only in an unconscious patient after the cervical spine has been cleared. To perform the doll’s eye examination, briskly rotate the patient’s head to the right and then to the left while holding the eyelids open and watching the eye movements. If the reflex is present (brainstem is intact), the patient’s eyes deviate away from the direction the head is rotated. Loss of brainstem integrity is presumed when eyes remain midline with rotation of the head or move in a disconjugate manner. The oculovestibular response (cold calorics) also assesses the integrity of the brainstem and is only evaluated in the unconscious patient. The head should be flexed to approximately 30 degrees, and 20 to 50 mL of cold saline is injected into the external auditory canal. Rapid nystagmus-like deviation of the eyes toward the irrigated ear is the normal response. No movement, disconjugate movement, or asymmetric movement indicates interruption in the functional connection between the medulla and midbrain. Severe dizziness and vomiting occur with this test in a conscious patient, so the ice water test is contraindicated in semiconscious or conscious patients. Another contraindication is tympanic membrane rupture.
A patient’s motor examination includes assessment for strength and symmetry when possible. Bilateral extremities should be assessed at the same time for comparison and to identify subtle abnormalities or differences. Refer to Chapter 22 for a more detailed description of the motor examination. A central noxious stimulus should be used to elicit a motor response in the uncooperative or unconscious patient. Central stimulation (e.g., trapezius pinch or sternal rub) produces an overall body response. Peripheral stimulation (e.g., nail bed pressure) is also important to assess to differentiate between a spinal cord injury and brain or brainstem injury. Voluntary purposeful movement should be distinguished from abnormal posturing. Abnormal motor responses include inequality in movement and strength from side to side and posturing. Posturing may be spontaneous or elicited by verbal or painful stimuli. Abnormal flexion posturing (previously called decorticate posturing) is rigid flexion with arms flexed toward the core and lower extremities extended. This type of posturing is associated with lesions above the midbrain. Abnormal extension posturing (previously called decerebrate posturing) is rigid extension of the arms with wrist flexion and rigid extension of lower extremities and is associated with an insult to the brainstem. Figure 21-6 illustrates abnormal flexion and extension posturing. Lateralization occurs when patients with TBI present with unilateral abnormal motor posturing. In a patient with hemiparesis contralateral to a fixed and dilated pupil, herniation should be suspected. 2. and 6.
![]() |
FIGURE 21-6 Abnormal. A, Flexion. and B, Extension. (Modified from Urden LD, Stacy KM, Lough ME: Thelan’s critical care nursing: diagnosis and management, ed 5, St. Louis, 2006, Mosby.) |
A detailed CN examination may be delayed until the secondary or focused survey but should be completed in all patients who are awake and can cooperate. In the severely injured patient the examination may be limited to pupillary responses (CN III), and corneal (CN V and VII) and gag reflexes (CN X).
Assessment of vital signs is an integral part of every initial assessment of a trauma patient. In the patient with brain injury, because of the significant influence the brain and brainstem have on cardiac and respiratory functions, changes in heart rate, blood pressure, and ventilatory rate may be indicators of neurologic deterioration. After major trauma and brain injury the body frequently is in a hyperdynamic state. As ICP rises, the body’s compensatory response is to increase systemic pressure in an attempt to maintain CPP. Hypertension is a common manifestation of severe brain injury. Increased heart rate and cardiac output are also part of the body’s compensatory response. There are a variety of cardiac dysrhythmias associated with traumatic brain injuries. Bundle branch blocks and atrial fibrillation may be seen with contusions, atrial and ventricular ectopy with subdural hematomas, and ST and T-wave changes with severe brain injuries. 7 Along with ventilatory rate, the pattern of breathing, work of breathing, and auscultation of breath sounds should be assessed. Respiratory pattern changes are common after severe brain injury and can assist in determining the level of brainstem dysfunction (Table 21-4). 2. and 8.
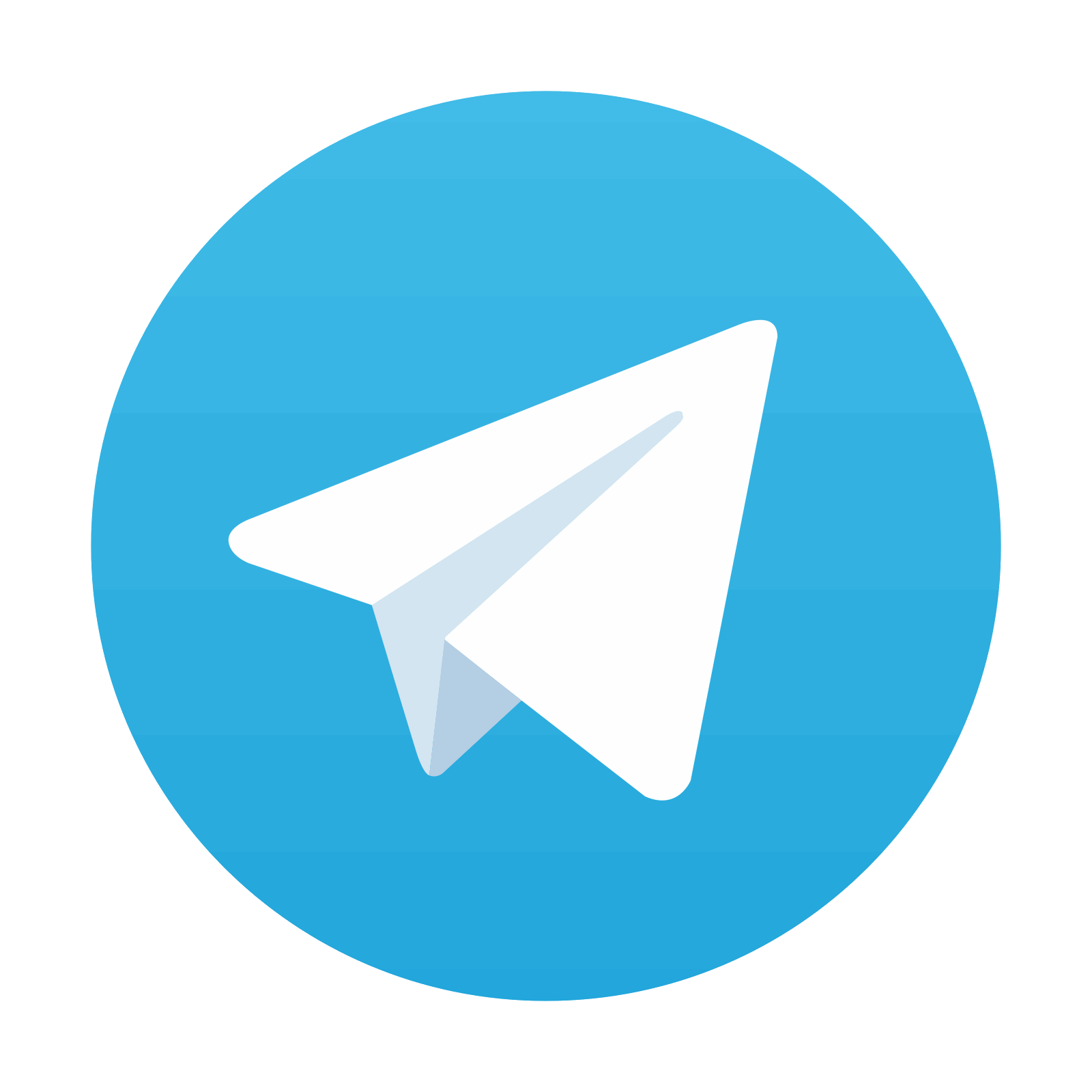
Stay updated, free articles. Join our Telegram channel

Full access? Get Clinical Tree
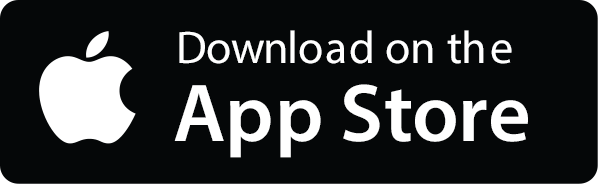
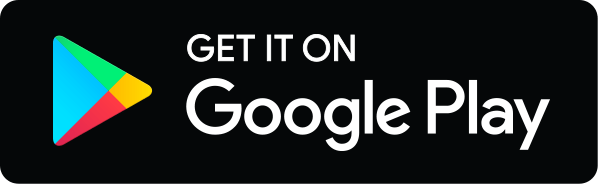
