- Iron metabolism and role of iron in red blood cell function
- Dietary source of vitamins B12 and folate
- Gastrointestinal absorption of vitamins B12 and folate
- Vitamin B12 and red cell production
- Causes of iron deficiency
- Causes of vitamin B12/folate deficiency
- How blood tests are used to diagnose iron deficiency anaemia
- How blood tests are used to diagnose anaemia caused by deficiency of vitamin B12 and/or folate
The means by which the results of full blood count (FBC) identify those patients who are anaemic was discussed in Chapter 15. It was emphasised that anaemia is not a single disease, but rather a syndrome with many possible causes. Successful treatment of anaemia depends crucially on identifying its cause. It will be remembered that tests included in the full blood count (most notably the MCV) suggest possible causes of anaemia, but further testing is necessary. The principle use of the five tests described in this chapter is to confirm the cause of anaemia.
Deficiency of iron is the most common cause of anaemia in the UK and around the world. World Health Organisation (WHO) best estimates suggest that 1.62 billion people are anaemic, that is 25% of the world population, and iron deficiency is the cause in around half of these cases1. In developed countries like the UK around 2–5% of the adult (non-pregnant) population have iron deficiency anaemia2. Prevalence is higher among pregnant women.
Measurement of the serum or plasma concentration of serum iron, total iron binding capacity (TIBC) and ferritin together provide the means of confirming a diagnosis of iron deficiency anaemia. Similarly measurement of the serum or plasma concentration of the vitamins B12 and folate provides the means of identifying those patients who are suffering so called megaloblastic anaemia. As we shall see anaemia is not the only condition in which abnormality in results of these five tests can be expected.
Normal physiology
Function of iron and iron metabolism
The oxygen delivery function of haemoglobin (Hb) contained within red cells is dependent on the presence of iron in the haem part of haemoglobin (see Chapter 15, Figure 15.3). Oxygen forms a reversible weak link with the single atom of iron in each of the four haem groups contained in the Hb molecule. Iron is thus essential for Hb production and function. The muscle protein, myoglobin and the function of some enzymes are also dependent on iron, although compared with the role of iron in Hb, these are of much less clinical significance.
Around 70% of the approximately 4–5 g of iron present in the body is contained in the Hb in circulating red cells (see Chapter 18, Figure 18.1). A little is contained in another much less abundant oxygen carrying protein called myoglobin, but most of the rest is stored in tissues (principally the liver but also the spleen and bone marrow). In these storage ‘compartments’ iron is contained within the proteins ferritin and haemosiderin. Some ferritin is present in the plasma part of blood and the concentration of ferritin in plasma is a reliable indicator of the body’s total iron tissue stores. Just 3–4 mg (i.e. 0.1% of total body iron) circulates in blood plasma, where it is bound to the transport protein transferrin. It is the concentration of this transferrin bound fraction of total body iron that is measured in the serum iron test.
Iron is well conserved by the body. When red cells die at the end of their 120 day life span, the iron they contain is returned via blood to body stores for bone marrow production of new red cells. Since iron is protein bound and therefore cannot be filtered from blood at the glomerulus, very little iron is excreted in urine; the only significant loss is that contained in surface epithelial cells constantly shed from the body; just 1 mg/day is lost from the body via this route. Since most of the body’s iron is contained within red cells, blood loss represents a potential route for significant iron depletion. For example, normal menstruation is associated with a loss of around 15 mg of iron every month. When this is taken into account, healthy pre-menopausal women lose on average 1.5–2 mg of iron a day. To replace these minimal losses and maintain normal iron stores, at least 1 mg of iron in the case of healthy children, adult men and post-menopausal women, and up to double this in the case of pre-menopausal women, must be absorbed every day from diet. In fact a normal well balanced diet contains approximately 10–15 mg of iron/day. The principal sources of dietary iron are meat (particularly red meat) and fish, green leafed vegetables and breakfast cereals. Vitamin C increases the availability for absorption of the iron present in vegetables and cereals. Absorption of dietary iron occurs in the upper small intestine (duodenum). Just 10% of available dietary iron is normally absorbed, sufficient to replace daily losses. It is vital for good health that iron stores remain replete but not overloaded with iron because, as we shall see, too much iron can be as damaging to health as too little iron. Since there is no control of the iron lost from the body, control of iron body stores depends crucially on the control mechanisms of dietary iron absorption. Absorption of dietary iron is minutely adjusted to meet the body’s requirements at the time. Hepcidin, a hormone synthesised in the liver is the key regulator of this dietary iron absorption. Excess dietary iron is not absorbed and passes from the body in faeces.
Function and metabolism of vitamins B12 and folate
Vitamins are a group of organic substances of widely differing chemical structure that are essential, albeit in tiny amounts, for life. They cannot be synthesised by the human body (vitamin D is an exception) and our only source is the food we eat. Most vitamins of the B group, which includes both B12 (alternative name cobalamin) and folate (alternative name folic acid), function as essential co-enzymes or co-factors in the enzymic reactions of cellular metabolism.
Specifically, B12 and folate are both required for the action of key enzymes involved in the synthesis of deoxyribonucleic acid (DNA) during cell division. Tissues characterised by rapid cell turn over and consequent unremitting cell division are particularly dependent on intact DNA synthesis and therefore vitamins B12 and folate. Bone marrow is one such tissue: blood cell production by the bone marrow continues minute by minute throughout life and an adequate supply of B12 and folate is essential for the continuing normal production of blood cells. Although essential for several other cellular enzyme reactions, the role of B12 and folate in the cell division required for normal production of blood cells is the one that is of prime clinical significance.
Both B12 and folate are naturally synthesised only by bacteria, and we obtain them by eating animal and plant foods that are naturally contaminated with these bacteria. The principal dietary source of vitamin B12 is meat (liver is a particularly rich source), fish and dairy products. Vegetables do not contain B12. Folic acid is present in green leafy vegetables; liver is a rich source. Most breakfast cereals and a few other processed foods are fortified with B12 and/or folate. The minimum adult daily requirement for B12 is 1.5 µg and for folate 200 µg. A normal healthy diet provides well in excess of the minimum requirement of B12, but only just twice the amount of folate required.
The acidity of gastric juice is vital for release of B12 from foods in the stomach prior to absorption lower down the gastrointestinal tract. Additionally, for absorption to occur B12 must first be bound to so called intrinsic factor, a peptide (small protein) produced by the gastric parietal cells. Thus functioning gastric parietal cells that line the stomach wall and produce both the hydrochloric acid and intrinsic factor present in gastric juice are essential to absorption of B12. Once bound to intrinsic factor, B12 is absorbed to blood via cells that line the lower (distal) end of the small intestine (the ileum). Absorbed B12 is transported in blood to bone marrow and other cells, bound to the transport protein, transcobalamin. The body has considerable capacity to store large reserves of vitamin B12 in the liver, sufficient in fact to remain in normal health for several years on an entirely vitamin B12-free diet.
Absorption of folate
Absorption of folate is less complex. It is absorbed higher up the small intestine than B12 at the jejenum and is transported in blood to bone marrow and other folate requiring tissues, either in its free form or bound to albumin.
Like vitamin B12, folate is stored principally in the liver. However folate stores are sufficient to last only a few months on a folate-free diet.
Normal production of red cells in the bone marrow is dependent then on:
- A healthy diet containing sufficient B12 and folate.
- Production of gastric acid and intrinsic factor by the stomach, for absorption of B12.
- Normal absorption at the ileum (i.e. functioning gastrointestinal tract).
- Adequate production of the transport protein transcobalamin.
Figure 18.2 Absorption of vitamin B12.
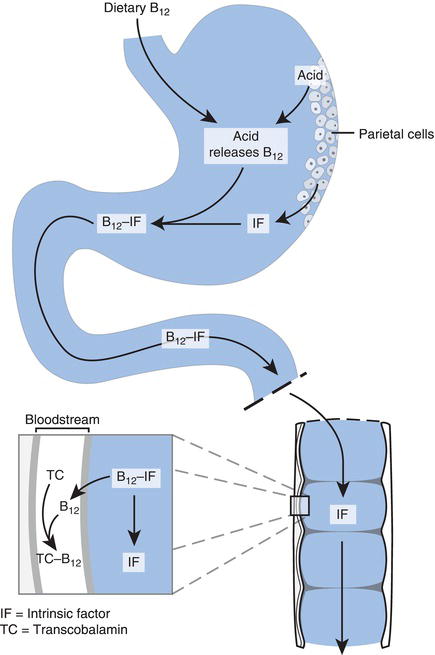
Laboratory measurement
With few exceptions the tests described in this chapter should be reserved for those patients in whom anaemia has been demonstrated by FBC (see Chapter 15). The mean cell volume (MCV) result indicates which of these tests are most appropriate. A reduced MCV indicates probable iron deficiency anaemia, in which case serum iron, total iron binding capacity and serum ferritin are appropriate. If MCV is raised then B12 and folate should be measured first.
What is being measured
Serum/plasma iron – this is the concentration of the small proportion of total body iron that circulates in the plasma part of blood bound to transferrin; it does not include the iron contained in red cells or that contained in ferritin.
Total iron binding capacity (TIBC) – this is a test performed on plasma or serum and is essentially a measure of the amount of transferrin available for iron to bind to. Remember, all the iron being transported in blood around the body is bound to transferrin.
Serum ferritin – ferritin is the protein in which iron is stored in tissues; a very small proportion escapes from tissues to blood. The concentration of ferritin in blood serum is a reliable indicator of total iron stores.
Serum B12 and folate – the concentration of vitamin B12 and folate in serum. A low result indicates deficiency.
Red cell folate – concentration of folate in red cells. A low result indicates deficiency.
Patient preparation
No particular patient preparation is necessary.
Timing of sample
No particular timing is required. It is best practice to collect blood at a time to coincide with routine transport to the laboratory.
Sample requirements
Around 5 ml of venous blood is sufficient for iron, TIBC and ferritin. Most commonly serum is used, in which case blood should be collected into a plain tube containing no anticoagulant. Blood for red cell folate must be collected into a tube containing the anticoagulant EDTA (lavender coloured top) bottle. A further 5 ml of venous blood is required for serum B12 and folate (collected into a plain tube containing no additive).
Interpretation of results
Approximate reference ranges
Serum Iron | 10–30 µmol/L |
Serum TIBC | 40–75 µmol/L |
Serum ferritin | 10–300 µg/L |
Serum B12 | of the order 150–1000 ng/L but values vary according to methodology; consult local laboratory |
Serum Folate | of the order 150–700 µg/L but values vary; consult local laboratory |
Conditions associated with abnormal results of either serum iron, TIBC or ferritin
These are:
- Iron deficiency anaemia.
- Chronic infection, inflammation malignancy (anaemia of chronic disease, ACD).
- Iron overload.
Iron deficiency anaemia
Causes
In particular patients there may be more than one cause: iron deficiency anaemia is frequently multifactorial. The many possible causes can be addressed under the four main headings:
- Insufficient iron in the diet, poor nutrition.
- Increased loss of iron (in blood) from the body.
- Inadequate absorption of dietary iron.
- Increased demand for iron.
It is unusual for poor diet to be the sole cause of iron deficiency in developed countries, although a diet relatively deficient of iron (e.g. high proportion of ‘junk’ food) may be a contributory factor in some cases. Poor nutrition is however a major cause of iron deficiency in most areas of the developing world.
The most common cause among adults in developed countries like the UK is chronic blood loss. Red cells contain 70% of the body’s iron, and blood loss represents a significant iron deficit. So long as iron tissue stores are replete, a single acute episode of even severe blood loss will not cause iron deficiency. However chronic blood loss, that is the continuous or regular loss of small amounts of blood over a prolonged period, will slowly exhaust iron stores. Chronic excessive menstrual blood loss (menorrhagia) is the most frequent cause of iron deficiency anaemia among non-pregnant, pre-menopausal women.
Chronic blood loss from the gastrointestinal tract, which may go unrecognised for many months or even years, as blood is lost imperceptibly in faeces, is a feature of a number of relatively common but serious diseases of the gastrointestinal tract. These include ulcerative colitis; cancer of oesophagus, stomach and colon; and stomach ulcers. Aspirin and some other non-steroidal anti-inflammatory drugs irritate the gastric lining of the stomach sufficiently to cause low grade bleeding in some people. For this reason long-term aspirin use is associated with a risk of iron deficiency anaemia. Together these gastrointestinal conditions associated with chronic blood loss account for most cases of iron deficiency anaemia among adult males and post-menopausal women. Some diseases of the gastrointestinal tract cause iron deficiency by reducing absorption of dietary iron. Foremost among these is coeliac disease (gluten sensitivity). This is an autoimmune disease, confined to genetically predisposed individuals, that is provoked by eating foods containing gluten (a protein constituent of wheat). It results in inflammatory damage to the mucosal lining of the small intestine that impairs absorption of many diet derived nutrients, including iron. Coeliac disease is common (affects 1–2% of the population) but currently remains under diagnosed. Iron deficiency anaemia can be a presenting sign, and coeliac disease has in recent years emerged as one of the more common causes of iron deficiency anaemia. A gluten-free diet (the standard treatment for coeliac disease) is effective in eradicating iron deficiency anaemia in such cases. Chronic inflammatory bowel conditions (Crohn’s disease and ulcerative colitis) are also associated with reduced iron absorption and consequent high risk of iron deficiency anaemia. Surgical removal of the stomach (gastrectomy) is associated with reduced absorption of iron.
Colonisation of the stomach with the bacterium Helicobacter pylori is very common, and usually benign. In some people however it leads to gastritis and gastric ulcers. In others it can cause iron deficiency anaemia. The mechanism is unclear but reduced absorption of iron is likely. Antibiotic treatment aimed at eradication of Helicobacter pylori might be an effective treatment for iron deficiency anaemia in these cases3.
Increased red cell production is one of the many physiological changes that occur during pregnancy. To accommodate this around two to three times the normal amount of iron is required. This increased demand for iron can lead to iron deficiency if iron stores are not replete, and iron deficiency anaemia is a not uncommon complication of late pregnancy4. Increased demand for iron during growth contributes to the iron deficiency that can occur in malnourished babies and children.
In all cases of iron deficiency anaemia, a cause should be sought no matter what the severity because it may be a presenting sign of serious underlying gastrointestinal or gynaecological disease. The advice of recently published guidelines2 is that all patients with unexplained iron deficiency anaemia, no matter what the severity, should be screened for coeliac disease using a blood test (serum IgA-tTG) that detects a causative autoantibody (the tissue transglutaminase antibody).
All those with unexplained iron deficiency anaemia should also have urine tested for the presence of blood (haematuria), a signal of possible bladder cancer. In addition upper and lower gastrointestinal tract investigation (e.g. barium meal, endoscopic examinations) should be considered for all male patients and all female patients over the age of 50 (post-menopausal) to identify disease associated with chronic gastrointestinal bleeding or inflammation that might explain the anaemia.
Symptoms
In addition to the general symptoms of anaemia already listed, patients with iron deficiency anaemia may exhibit some other specific symptoms of iron deficiency which include:
- Glossitis (inflammation of the tongue).
- Angular cheilosis (ulceration of lips at the corners of the mouth).
- Changes to nails (unusually brittle; may become spoon shaped – indented (koilonychia)).
Results of blood testing
Serum ferritin concentration reflects iron stores, so that a reduction in serum ferritin is evident before anaemia develops, during the period of progressive iron store depletion. By the time that iron stores are totally depleted, when signs and symptoms of iron deficiency anaemia begin to occur, serum ferritin concentration is extremely low or undetectable. Serum iron is usually reduced, but may remain at the low end of the reference range. Serum total iron binding capacity is always raised.
Typical blood results in iron deficiency anaemia are:
- Serum iron usually reduced, in the range 5–10 µmol/L (may be at the low end of the reference range).
- Serum TIBC raised (i.e. greater than 75 µmol/L).
- Serum ferritin greatly reduced (usually undetectable, i.e. <5 µg/L).
Chronic inflammation and infection
As outlined in Chapter 15, many patients with chronic infectious or inflammatory disease as well as those with malignant disease may become mildly to moderately anaemic (it is unusual for Hb to fall lower than 9 g/dl in such cases).
This is called anaemia of chronic disease (ACD). Although ACD is not the result of iron deficiency (iron stores are not depleted), it is associated with disturbance of iron metabolism that is reflected in blood test results. Serum iron concentration is usually reduced, as it is in iron deficiency anaemia. However in contrast to iron deficiency anaemia, serum TIBC is reduced and serum ferritin is either normal or in some cases increased. Since a deficiency of iron is not the problem, iron supplements are of little use to these patients. Indeed such treatment may be harmful by putting them at risk of iron overload. Instead treatment is directed at the underlying condition; as this resolves, or is ameliorated anaemia may itself lessen. Other treatment options include recombinant erythropoietin injections.
To summarise, patients with chronic infections (e.g. TB, bacterial endocarditis, pneumonia etc.) and chronic inflammation (e.g. rheumatoid arthritis, SLE, Crohn’s disease etc.) as well as some cancer patients may be anaemic. The severity of anaemia reflects the severity of the underlying disorder.
ACD is associated with:
- Reduced serum iron concentration.
- Reduced serum TIBC.
- Normal or raised serum ferritin concentration.
Iron overload
In the physiological state all iron in the body is bound to a protein (Hb in red cells, ferritin in storage compartments and transferrin in blood plasma). In this state it is non-toxic. However free (unbound) iron is toxic. There is a finite amount of each of the ‘protective’ binding and storage proteins, and once they are saturated with iron, any additional iron in cells exists in its toxic-free (unbound to protein) state. Free iron is toxic because it promotes production of highly reactive free radicals that disrupt normal cell structure and function. Unchecked this can lead to tissue damage. Tissues affected in disease caused by chronic iron overload include:
- Pancreas – fibrosis of pancreas leading to diabetes.
- Liver – cirrhosis and eventual liver failure, liver cancer.
- Heart – arrhythmias, heart failure.
- Gonads – impotence.
- Joints – severe joint pain (similar to arthritis).
Causes
Hereditary haemachromatosis. Those with this genetically determined disease absorb increased amounts of available iron in diet, 3–4 mg/day instead of the normal 1–2 mg. There is no physiological means of increasing iron excretion so iron accumulates at the rate of 0.5–1.0 g/year. In late middle age when first clinical effects of iron overload occur, total body iron may be in the range 20–40 g rather than normal 4–5 g. Around one in ten of us carry a single copy of the defective gene (it is the most common inherited genetic defect). Only inheritance of the defective gene from both parents (i.e. two copies of the defective gene) results in the most severe form of haemachromatosis, which leads to diabetes, cirrhosis etc. Other causes of iron overload include repeated blood transfusion and inappropriate iron therapy. One unit of blood contains around 250 mg of iron in red cells, 100 times the daily requirement. Patients whose treatment includes repeated blood transfusion over a prolonged period (e.g. those suffering thalassaemia and sickle cell disease) are particularly at risk of iron overload.
Blood test results
The blood test results of those with iron overload are, as might be expected, the opposite of those associated with iron deficiency. No matter what the cause, iron overload is associated with:
- Raised serum iron.
- Reduced serum TIBC.
- Raised serum ferritin.
Conditions associated with reduced B12 and folate results
Megaloblastic anaemia
Megaloblastic anaemia is a term applied to all those anaemias in which due to impaired DNA synthesis there is abnormal development of blood cells in the bone marrow. Impaired DNA synthesis results in production of large (mega) immature (blast) red cells, many of which do not develop to maturity and die within the bone marrow. There is thus a marked reduction in the number of circulating red cells, hence the anaemia. Some of those red cells that do survive and appear in peripheral blood are larger than normal, often characteristically oval in shape. Large red cells in peripheral blood are called macrocytes, so the term macrocytic anaemia is sometimes used as an alternative name for megaloblastic anaemia, although the terms are not, strictly speaking, synonomous. The effect of impaired DNA synthesis is not confined to red cells, numbers of both white cells and platelets may be reduced in those with severe megaloblastic anaemia. With a few very rare exceptions, the cause of the impaired DNA synthesis that leads to megaloblastic anaemia is vitamin B12 and/or folate deficiency.
Causes of vitamin B12 or folate deficiency
The causes can be summarised under the following headings:
- Dietary deficiency.
- Failure to absorb B12 due to inadequate production of intrinsic factor.
- Failure to absorb either B12 or folate due to gastrointestinal disease.
- Increased demand for B12 and folate during pregnancy.
Dietary deficiency of B12 is rare except among strict vegetarians (vegans) who eat no dairy products. Dietary deficiency of folate is more common; in fact it is the most common cause of folate deficiency. Unlike vitamin B12, folate in food can be destroyed by cooking, and the normal dietary intake is close to the minimum required. Dietary deficiency of folate is quite common among chronic alcoholics; this combined with effects of alcohol on folate metabolism, render this group particularly susceptible to folate deficiency. The infirm elderly, whose diet is often not well balanced and may be deficient of folate, are considered another group at risk of dietary deficiency of folate.
The most common cause of vitamin B12 deficiency is failure to absorb the vitamin due to lack of intrinsic factor. The specific type of megaloblastic anaemia that results from lack of intrinsic factor is known as pernicious anaemia. Those with pernicious anaemia are unable to produce intrinsic factor because of damage (autoimmune in nature) to the parietal cells of the stomach lining, where intrinsic factor is produced. The condition tends to run in families and is often associated with other autoimmune diseases (e.g. thyroid disease, Addison’s disease, coeliac disease and Type 1 diabetes). Absorption of folate is not affected in pernicious anaemia. Patients with pernicious anaemia must be given B12 by injection, as that given by the oral route cannot be absorbed.
Partial and total gastrectomy can of course also result in reduced production of intrinsic factor.
Various gastrointestinal diseases associated with inflammatory or other damage to areas where B12 and folate are normally absorbed can result in reduced absorption and vitamin deficiency. Surgical removal of areas of the gastrointestinal tract (e.g. jejunal or ileal resection) can lead to either B12 or folate deficiency. Patients receiving such surgery may be given regular injections of B12 and folate to prevent anaemia developing. Pregnancy is associated with increased demand for B12 and folate. A deficiency at around the time of conception and during the first weeks of pregnancy is associated with increased risk of giving birth to a baby with serious neural tube defects such as spina bifida. This provides the rationale for the well-publicised advice to women contemplating pregnancy and newly pregnant women to take folic acid supplements.
Symptoms of megaloblastic anaemia
Whether they are caused by dietary deficiency or poor absorption of B12 or folate, the resulting symptoms may include:
- General symptoms of all anaemias.
- Mild jaundice.
- Glossitis (inflamed tongue).
- Angular cheilosis (sores at the corner of the mouth).
Patients with B12 deficiency, but less commonly those with folate deficiency, may also suffer a progressive condition of the nervous system called sub-acute combined degeneration of the cord. Those with this additional effect of B12 deficiency may present with any of a range of neurological/cognitive symptoms including:
- Paraesthesia (abnormal sensation, e.g. numbness, tingling in fingers and toes).
- Gait ataxia, resulting in difficulty in walking.
- Increased irritability.
- Memory loss.
- Depression.
- Rarely, personality change and other overt psychiatric problems.
These neurological/psychiatric symptoms of B12 deficiency may be present with or without signs and symptoms of anaemia.
Blood test results
Serum B12 is usually reduced in pernicious anaemia and all other cases of megaloblastic anaemia and neuropathy caused by deficiency of B12. In some cases the serum level remains at the low end of the reference range, causing some diagnostic difficulty. Serum folate is usually normal but may be slightly raised. Red cell folate by contrast is either normal or low in cases of B12 deficiency.
In cases of megaloblastic anaemia caused solely by folate deficiency, serum B12 is normal, but as might be expected both serum and red cell folate are reduced. Red cell folate is considered a more reliable test of folate status because serum folate may be low in some illnesses (e.g. severe liver and kidney disease) despite normal folate status.
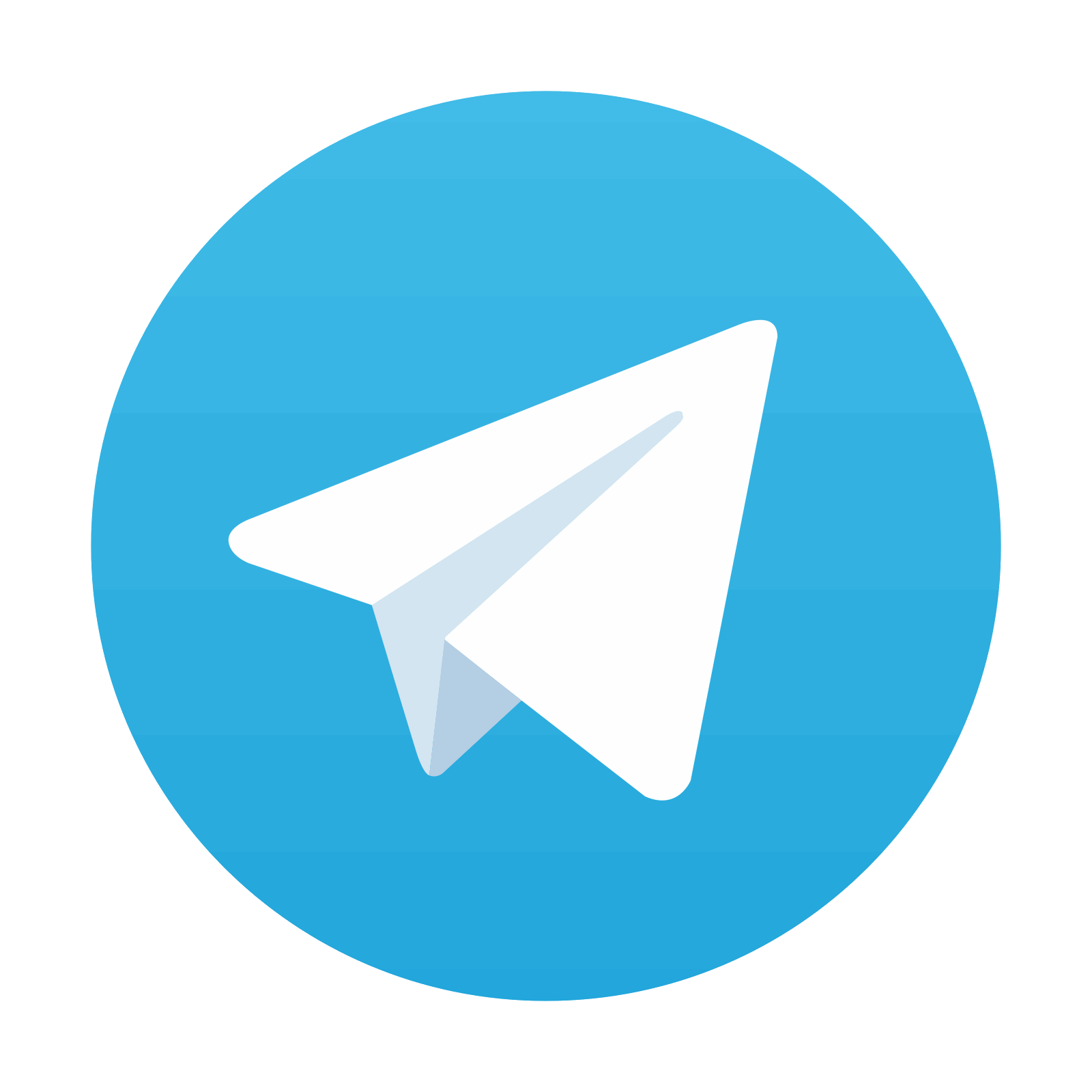
Stay updated, free articles. Join our Telegram channel

Full access? Get Clinical Tree
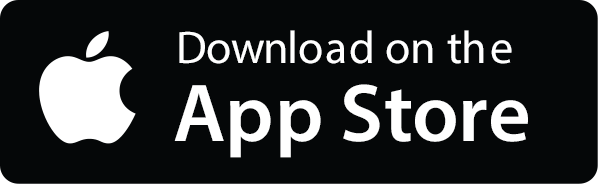
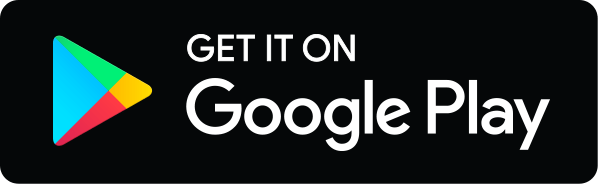