- Production, function and fate of red blood cells (erythrocytes)
- Structure and function of haemoglobin
- Defining red cell indices – haematocrit (PCV), MCV and MCHC
- How patient haemoglobin level is used to confirm anaemia
- Principle causes and consequences of anaemia
- How red cell indices help in establishing cause of anaemia
Of all laboratory blood tests, the full blood count (FBC) is the most frequently requested, reflecting the wide range of both common and less common disturbances of health that may be associated with abnormality in FBC results. It is not one test, but a panel of tests including a count of each of the three cellular or formed elements of blood: red cells (erythrocytes), white cells (leucocytes) and platelets (thrombocytes). In this first of two chapters that focus on the FBC, we consider the red cell count and several other tests included in the FBC which all relate to red cell function. All these tests (listed in Table 15.1) are used primarily to identify those patients who are anaemic; they also help in elucidating the cause of that anaemia. In Chapter 16 we consider the significance of the total and differential white cell count, whilst the platelet count will be dealt with in Chapter 17, which focuses on tests of blood coagulation.
Blood film
For this test blood is spread in a thin film on a glass microscope slide, then stained with dyes and examined under the microscope. A blood film report includes the details of any abnormalities in appearance and size of red cells. A blood film is usually only prepared and examined if results of the tests in Table 15.1 are abnormal.
Table 15.1 Tests included in a full blood count (FBC) that relate to red blood cells.
Name of test (common abbreviation) | What is measured | Unit of measurement |
Red blood cell (RBC) count | The number of red blood cells | Number of thousand million (i.e. 109)red cells in 1 litre of blood (109/L) |
Normal physiology
Red cell (erythrocyte) production
Red cells are the most abundant of the three formed elements in blood outnumbering leucocytes (white cells) by around 1000:1 and platelets by 100:1. The process of blood cell production, called haemopoiesis, takes place within the bone marrow. During infancy the bone marrow of all bones have the capacity to manufacture blood cells, but in adulthood this is limited to the bone marrow within the vertebrae, ribs, sternum, skull and pelvis as well as the ends of the long bones, femur and humerus. All blood cells are derived from bone marrow stem cells, which have the potential to differentiate to cells committed to becoming either mature red cells, white cells or platelets. The most primitive of those cells within bone marrow that are destined to become mature red cells is the pro-normoblast (alternative name pro-erythroblast). The pro-normoblast develops by cell division and differentiation through recognisable stages to normoblast (alternative name erythroblast), reticulocyte and finally the mature red cell or erythrocyte (Figure 15.1). This development from stem cell to mature red cell is called erythropoiesis and is characterised by:
- Gradual reduction in cell size.
- Loss of nucleus and therefore ability to divide.
- Loss of internal cell organelles.
The final stage of maturation, reticulocyte to erythrocyte occurs both within the bone marrow and in peripheral blood; normally around 1–2% of the circulating red cell population is reticulocytes. No red cell more primitive than the reticulocyte is normally present in blood.
Mature red cells have a life span of around 120 days. Constant replacement is necessary; on average around 2.3 million red cells are produced every second by the bone marrow throughout life. This production is regulated by erythropoietin, a hormone synthesised in the cells of the kidney (Figure 15.2). In response to a decreasing blood oxygen level, the kidneys release erythropoietin to blood for transport to the bone marrow. Here erythropoietin stimulates erythropoiesis and thereby red cell production. As red cell numbers increase, the oxygen content of blood increases, and kidney production of erythropoietin is stepped down.
Structure and function of red cells
The structure of the mature red cell is well suited to its primary functions: transport of oxygen from the lungs to the tissues and transport of carbon dioxide from tissues to the lungs. Central to this function is haemoglobin, the protein contained within red blood cells. Haemoglobin production occurs within the red cell during its development in the bone marrow and is complete before full maturation. Each nearly mature red cell (reticulocyte) leaves the bone marrow with its full complement of 250–300 million molecules of haemoglobin.
Commonly described as a biconcave disc the mature red cell (erythrocyte) can be imagined as a flattened sphere with its sides pushed in. This singular shape allows the largest surface area for a given volume, providing maximum possible area for oxygen and carbon dioxide gas exchange.
The diameter of the red cell is around 8 µm, twice the diameter of the smallest blood vessels through which it must pass. The membrane is able to deform itself, altering the shape of the red blood cell, so that it can ‘squeeze’ through the microvasculature within tissues, where gases (oxygen and carbon dioxide) are exchanged between blood and tissue cells. Without a nucleus and other internal organelles, the mature erythrocyte may be regarded – albeit simplistically – as little more than a deformable membranous bag, stuffed full of haemoglobin.
Haemoglobin structure and function
Haemoglobin is the oxygen carrying protein pigment present in red cells, which gives blood its colour.
The haemoglobin molecule (Figure 15.3) comprises four folded chains of amino acids. These together form the protein or globin portion of the molecule. Each of the four globin sub-units has a much smaller haem group attached, and at the centre of each haem group is an atom of iron in the ferrous state (Fe2+). Whilst the structure of the haem group is always the same, the exact sequence of amino acids in the globin sub-units varies slightly, giving rise to four possible globin chains: alpha (α), beta (β), gamma (γ) and delta (δ). Around 97% of adult haemoglobin is haemoglobin A (HbA) comprising 2 α and 2 β globin sub-units. The remaining 3% is HbA2 (2 α and 2 δ globins). In the developing foetus and for the first few months of life, foetal haemoglobin (HbF) is the only haemoglobin produced; HbF is composed of 2 α and 2 γ globin sub-units. The structure of haemoglobin is of more than passing academic interest. There are a large group of inherited disorders of haemoglobin synthesis and structure, collectively known as the haemoglobinopathies. Most are rare, but one, sickle cell disease is relatively common and therefore warrants special attention; they are discussed in Chapter 23.
Haemoglobin transport of oxygen
A very small proportion of oxygen transported in blood is simply dissolved in blood plasma, but most (98–99%) is transported bound to haemoglobin. The necessary oxygen combining property of haemoglobin depends on the single atom of iron present at the centre of each of the four haem groups. An oxygen molecule forms a weak, reversible ionic link with each of these iron atoms in turn; the product of this reaction is oxyhaemoglobin. When all four haem groups are occupied with oxygen the haemoglobin molecule is said to be saturated. The affinity of haemoglobin for oxygen, that is the extent to which it is saturated with oxygen, depends on the amount or partial pressure (P) of oxygen dissolved in the blood plasma in which the red cells, containing haemoglobin are suspended. This is described graphically in the oxygen dissociation curve (see Chapter 7, Figure 7.2). It is clear from the graph in Figure 7.2 that haemoglobin becomes increasingly saturated with oxygen as the partial pressure of oxygen (PO2) increases. The physiological implications of this relationship are central to the oxygen binding and delivery function of haemoglobin. In the lungs, oxygen in inspired air diffuses from alveoli to blood plasma so that PO2 of blood here is high (around 95 mmHg). Since this relatively high PO2 is associated with high haemoglobin affinity for oxygen, haemoglobin quickly (within a few seconds) becomes almost 100% saturated with oxygen. Conversely in the tissues, blood PO2 is relatively low (only around 40 mmHg) and consequently haemoglobin here has less affinity for oxygen. As a result oxygen is released from haemoglobin and diffuses from blood to tissue cells, where it is required for cell metabolism.
Role of red cell and haemoglobin in the transport of CO2
Whilst the transport of oxygen from lungs to tissues is almost entirely due to haemoglobin in red cells, the transport of carbon dioxide in the reverse direction is slightly more complex. Carbon dioxide is more soluble in blood plasma than oxygen, so that a significant amount of carbon dioxide is transported simply dissolved in blood plasma. The remainder is transported in two other ways; both mechanisms involve carbon dioxide diffusing from plasma to red cells. Within red cells most carbon dioxide is converted to bicarbonate, and this bicarbonate diffuses from red cells to plasma for transport to the lungs. The rest remains in red cells loosely bound to the haemoglobin that has just released its oxygen to the tissues.
[Note: The oxygen and carbon dioxide transporting function of haemoglobin is also dealt with in a discussion of respiratory physiology contained in Chapter 7; readers might find it useful to refer to that discussion.]
Normal red cell destruction
After around 120 days circulating in peripheral blood, red cells are no longer viable and are removed from blood to the reticulo-endothelial (RE) system, as blood passes through the bone marrow, spleen and liver. In these RE sites, the defunct red cell is engulfed by, and internalised to (the process is called phagocytosis) a much larger cell called a macrophage, whose function is controlled destruction of the red cell. Within macrophages the red cell membrane is first degraded, releasing haemoglobin, which is split into its constituent parts: haem and globin. The iron present in haem is recylced for production of new red cells and globin chains are broken down to amino acids, which enter the amino acid pool for synthesis of new proteins. What remains of haem after removal of iron is converted to the yellow pigment bilirubin. This is transported in blood to the liver for further metabolism and eventual excretion, mostly via bile in faeces; a little is excreted as the bilirubin metabolites, urobilin and urobilinogen, in urine.
In health the rate of red cell production in bone marrow matches the rate of red cell destruction in the reticuloendothelial system, so that the number of red cells circulating in peripheral blood remains broadly constant and sufficient to provide all tissue cells with the oxygen they require to survive and function.
Laboratory measurement of FBC
Patient preparation
No particular patient preparation is necessary.
Timing of sample
There are no special timing requirements so blood is best sampled at a time that coinicides with routine transport to the laboratory. Blood for FBC should not be stored longer than 12 hours before transport to the laboratory. FBC is one of those tests that can be performed urgently and out of normal laboratory hours, if necessary. In such circumstances it is essential to telephone the laboratory and transport the blood immediately it has been sampled.
Type of sample
Venous blood is preferable, ideally collected without the use of a tourniquet (if used, a tourniquet should not be left for more than a minute or two before blood is sampled). Capillary blood collection is appropriate if venous blood collection poses difficulty (e.g. in babies and those with ‘difficult’ veins).
Blood collection bottle
Blood for FBC must be collected into a special tube (usually lavender or pink coloured top) which contains the anticoagulant, K+EDTA. This both prevents the blood from clotting and preserves the structure of blood cells.
Sample volume
The sample bottles for FBC have a ‘line to fill’ marked on the label. This is usually 2.5 ml, or 0.5 ml in the case of paediatric sized bottles for capillary blood sampling. This volume will result in the correct ratio of blood to anticoagulant. It is important for accurate result that neither too much nor too little blood is added to these tubes and that anticoagulant and blood are mixed by gentle inversion, as soon as the blood is sampled. Inadequate mixing can result in formation of tiny blood clots, and inaccurate results.
Interpretation of Hb RBC and red cell indices results
Approximate reference ranges
Red blood cell (RBC)
Adult Males | 4.5 – 6.5 × 1012/L |
Adult Females | 3.9 – 5.6 × 1012/L |
At birth | 3.5 – 6.7 × 1012/L |
Childhood | 4.1 – 5.3 × 1012/L |
Haemoglobin (Hb)
Adult Males | 13.5 – 17.5 g/dl |
Adult Females | 11.5 – 15.5 g/dl |
At birth | 14.0 – 24.0 g/dl |
Childhood | 11.0 – 14.0 g/dl |
[Note: In some laboratories and clinical texts the unit of haemoglobin measurement is grams per litre (g/L) rather than grams per deciliter (g/dl). To convert g/L to g/dl, divide by 10. Thus Hb 135 g/L is the same as Hb 13.5g/dl.]
Packed cell volume (PCV) or Haematocrit (Ht)
Males | 40 – 52% |
Females | 36 – 48% |
Mean cell volume (MCV)
Adults | 80 – 95 fl |
At birth | 100 – 135 fl |
Childhood | 71 – 88 fl |
Mean cell haemoglobin concentration (MCHC) – 20 –35 g/dl
Red (cell) distribution width (RDW) – 10 –15%
Critical values
Haemoglobin | <7.0 g/dl or >20.0 g/dl |
PCV (Haematocrit) | <20% or >60% |
Terms used to describe red cells
Normocytosis | average size of red cell is normal. |
Microcytosis | average size of red cells is smaller than normal. |
Macrocytosis | average size of red cells is larger than normal. |
Anisocytosis | red cells vary in size more than normal. |
Poikilocytosis | red cells vary in shape. |
Normochromasia | red cells stain normally indicating they contain normal amount of haemoglobin. |
Hypochromasia | red cells appear weakly stained indicating they contain less haemoglobin than normal. |
Conditions associated with reduction in RBC, Hb, PCV(Ht)
Anaemia
Anaemia (literally, without blood) is the collection of signs and symptoms that result from reduced oxygen delivery to tissues, due to a decrease in the total number of red blood cells and consequent reduction in haemoglobin concentration of blood. There are many possible causes, so that anaemia is not a disease entity itself, but rather a sign of some underlying disease, which must be identified if treatment of anaemia is to be successful.
Of all the signs and symptoms that may be present in an anaemic patient, the constant sign that is usually used to define anaemia is reduced haemoglobin (Hb) concentration. Diagnosis of anaemia is established if Hb concentration is below the lower limit of the reference range, that is less than 13.5 g/dl for adult males and less than 11.5 g/dl for adult females. In children, who normally have slightly lower levels of Hb, a diagnosis of anaemia is made if Hb is less than 11.0 g/dl. The lower the Hb, the more severe is the anaemia, and anaemia is excluded by the finding of Hb concentration within the reference range (there is an important exception to this, to be explained).
Reduced red cell count and PCV is also a feature of anaemia, although the magnitude of the reduction of red cell count depends not only on the severity of the anaemia, but also its cause. The principle pathological effect of anaemia is reduced delivery of oxygen to tissue cells but the extent to which this causes symptoms, depends on several factors including:
- Severity of the anaemia: many patients with mild anaemia, i.e. Hb >10 g/dl have no symptoms, but severe anaemia (Hb <6 g/dl) is almost always associated with symptoms.
- Speed of onset: rapid onset of anaemia is more likely to result in symptoms than that which has developed slowly.
- Co-existing disease: the normal physiological (compensatory) response to anaemia that ensures, so far as is possible, continued delivery of oxygen to tissues despite reduced Hb concentration is dependent on functioning respiratory and cardiovascular systems. Those with co-existing respiratory and/or cardiovascular disease are particularly vulnerable to the effects of anaemia, and more likely to manifest symptoms.
Signs and symptoms of anaemia
To some extent signs and symptoms depend on the cause of anaemia but there are some that occur irrespective of cause. The principle generalised symptoms of anaemia include:
- Pallor.
- Tiredness and lethargy.
- Shortness of breath, particularly on exertion.
- Dizziness, fainting.
- Headaches.
- Increased heart rate (tachycardia), palpitations.
The absence of symptoms does not preclude anaemia; many mildly anaemic individuals remain asymptomatic, particularly if anaemia has developed slowly (chronically) and there has been time for induction of compensatory mechanisms that help to ensure oxygen delivery to tissues despite reduced oxygen carrying capacity of blood.
Causes of anaemia
Whilst Hb, RBC and PCV measurement all help in identifying those patients who are anaemic and in assessing the severity of anaemia, they provide little information about cause.
Anaemia may be caused by:
- Significant acute blood loss (haemorrhage), e.g. trauma, surgery, postpartum haemorrhage (PPH).
- Deficiency of iron (required for Hb production); this is the most common cause of anaemia and is discussed further in Chapter 18.
- Anaemia is a common complication of chronic inflammatory disease (e.g. rheumatoid arthritis), chronic infectious disease (e.g. tuberculosis) and malignant disease (cancer). Because there is a common mechanism (inflammation) that gives rise to the anaemia in all these conditions, it is called anaemia of chronic disease (ACD). ACD is the second most common cause of anaemia after iron deficiency, and the most common cause in the elderly. (The elderly represent the age group most likely to be suffering the underlying chronic/malignant diseases.) A distinguishing feature of ACD is that it is almost invariably mild in nature, with Hb rarely being less than around 9–10 g/dl.
- Deficiency of vitamins B12 and/or folate that are both required for production of red cells. Pernicious anaemia accounts for most cases of anaemia in this group, but the whole group is discussed further in Chapter 18.
- Chronic kidney disease (CKD). Inadequate production of erythropoietin (required for regulation of red cell production) is the main cause of the anaemia that commonly occurs in those with CKD.
- Some diseases are associated with an increased rate of red cell destruction, such that despite maximal red cell production by the bone marrow, the number of circulating red cells declines; anaemia is the inevitable consequence. Haemolytic anaemia, the name given to anaemia that results from increased red cell destruction, is a feature of a number of conditions including: sickle cell disease, transfusion reaction, haemolytic disease of the newborn, malaria and lead poisoning. Very rarely, haemolytic anaemia can be an adverse effect of prescribed drugs, most commonly the cephalosporins (a class of antibiotics). A distinguishing feature of haemolytic anaemia is that it can result in jaundice, a condition that is discussed in some detail in Chapter 11.
- Rarely, anaemia can result from damage to, or defect in, bone marrow stem cells and consequent reduced bone marrow production of red cells (and all other blood cells). This is called aplastic anaemia which can be due to an inherited defect, or much more commonly acquired as a result of exposure to radiation, some drugs, toxic chemicals or viruses. No cause can be identified in many cases.
- Reduced bone marrow production of red cells and consequent anaemia is a feature of malignant diseases of bone marrow such as leukaemia and myeloma.
- Prematurity is a major risk factor for anaemia in the neonatal period for two reasons: decreased red cell production due to inadequate erythropoietin; and decreased red cell survival (around 35 days rather than the normal 120 days). Additionally, very premature babies require frequent blood testing, which can represent a significant blood loss in the context of their very low total blood volume.
The red cell indices, MCV, MCHC, MCH and RDW, along with microscopic examination of red cells, help in identifying the cause of anaemia. Initially, when investigating the cause of anaemia, MCV is the most useful because all cases of anaemia can be classified according to the average size of red cell (MCV) to one of three groups:
- Microcytic anaemia (low MCV).
- Normocytic anaemia (normal MCV).
- Macrocytic anaemia (raised MCV).
The microcytic anaemias are those that result from iron deficiency and thalassaemia. Some patients whose anaemia is due to chronic disease have a microcytic anaemia, but this is not typical.
Most patients with ACD have a normocytic anaemia. Other causes of anaemia in this normocytic group include acute blood loss (haemorrhage); the haemolytic anaemias; anaemia caused by decreased erythropoietin production (CKD), anaemia caused by damage to bone marrow stem cells (aplastic anaemia) and anaemia, which results from malignant disease of the bone marrow (i.e. the leukaemias etc).
Macrocytic anaemias are confined to those that result from vitamin B12 or folate deficiency.
RDW is helpful in further distinguishing the cause within these three groups; so that, for example, iron deficiency and thalassaemia are both associated with microcytic anaemia. However iron deficiency is associated with a raised RDW, whereas RDW is normal in those with thalassaemia. Similarly RDW is able to distinguish different causes of normocytic anaemia. For example, anaemia of chronic disease is associated with normal RDW whereas haemolytic anaemias are associated with raised RDW. Simply by examining MCV and RDW results of anaemic patients then, it is possible to narrow down the likely cause of anaemia.
The examination of a stained blood smear under the microscope may also be useful because the shape and staining characteristics of red cells from an anaemic patient may give important clues as to cause. For example the red cells of patients with sickle cell anaemia can have a characteristic sickle shape, which gives the condition its name. Relatively small red cells that stain weakly (due to abnormally low concentration of haemoglobin) indicate possible iron deficiency whilst large cells, typically oval in shape indicate anaemia is probably due to deficiency of vitamin B12 or folate. Malarial parasites can be seen in the red cells of a patient with malaria associated anaemia, and anaemia caused by lead poisoning is often evident as abnormal staining of red cells (the abnormality is called basophilic stippling).
Causes of increased red cell count, haemoglobin and PCV (Ht)
Polycythaemia
Polycythaemia (literally many blood cells) is the opposite of anaemia. Increased red cell count and haemoglobin concentration is the hallmark of polycythaemia. Since PCV is dependent on the number of red cells, this too is raised in polycythaemia. Polycythaemia may arise as a response to any physiological or pathological condition in which blood contains less oxygen than normal. In response to a low blood oxygen level, the kidney increases erythropoietin production resulting in turn in increased red cell production. This so-called secondary polycythaemia is a feature of:
- Living at high altitude (where inspired air has relatively less oxygen).
- Cigarette smoking (carbon monoxide present in inhaled cigarette smoke binds to haemoglobin, displacing oxygen).
- Chronic lung disease (oxygen transfer from lungs to blood is compromised).
- Cyanotic heart disease (blood is less well oxygenated than normal due to congenital structural defects in the heart).
Primary polycythaemia or polycythaemia vera is a quite separate rare malignant disease of the bone marrow in which bone marrow stem cell proliferation results in marked over-production of red cells (white cell and platelet numbers are also often increased). This huge increase in red cell numbers increases the viscosity (fluidity) of blood and several of the signs and symptoms (headache, increased blood pressure) are related to this increased blood viscosity.
Effect of hydration on RBC, Hb and PCV(Ht)
Measured red cell count, haemoglobin and PCV are all affected by the state of hydration of the patient. Dehydration is associated with increased RBC Hb and PCV simply because plasma volume is reduced. The absolute number of red cells and amount of haemoglobin in blood however remains unchanged. Conversely a patient who has received too much fluid and is overhydrated will have a reduced Hb, red cell count and PCV. This effect of hydration has to be taken into account when interpreting results from patients who are either dehydrated or overhydrated. An increased plasma volume is a normal physiological effect of pregnancy so that pregnancy is associated with gradual decrease in Hb, PCV and RBC, even though absolute numbers of red cells and Hb are normal. Thus a pregnant woman with a slight reduction in Hb, RBC and PCV is not necessarily anaemic. In fact anaemia would not normally be diagnosed during pregnancy if Hb is greater than 10.5 g/dl.
Another anomaly that arises if Hb concentration is being used to define anaemia concerns acute blood loss (haemorrhage). This is associated with equivalent loss of both red cells (containing haemoglobin) and plasma. So despite what might be a significant loss of red cells and contained Hb, Hb concentration, red cell count (concentration) and PCV all remain, initially at least, normal. It is not until compensatory mechanisms (or fluid replacement therapy) have invoked restoration of fluid volume towards normal that anaemia becomes evident in FBC results of a patient who has suffered acute blood loss.
Other causes of a raised mcv
It is worth noting that MCV may be raised in patients who are not anaemic, that is have a normal Hb. The principal causes of an isolated increase in MCV are alcohol abuse and cirrhosis of the liver. An isolated raised MCV in a patient suspected of alcohol abuse is considered objective evidence of continued alcohol use.
The reticulocyte count
As previously discussed reticulocytes are immature red cells. For technical reasons and because it has limited clinical utility, a count of the number of reticulocytes in blood is not included in the routine FBC. It is however offered as a separate test by most laboratories. A brief discussion is appropriate here because its utility is confined to investigation of the anaemic patient – a major focus of this chapter. The sample requirement for reticulocyte count is the same as for FBC (as already discussed).
Approximate reference range
20–80 × 109/L
(Some laboratories report reticulocytes as a percentage (%) of the total red cell count in which case the approximate reference range is 0.5–2.0%.)
Interpretation of test result
The clinical value of the reticulocyte count rests on the observation that the normal physiological response to anaemia is to increase bone marrow production of red cells. This increased rate of red cell production is evident as an increased number of immature red cells (reticulocytes) being released to blood from bone marrow, and thereby an increased reticulocyte count. This effect of the physiological response to anaemia is referred to as reticulocytosis. Evidence of reticulocytosis (i.e. increased reticulocyte count) in an anaemic patient implies that the anaemia is not the result of defect in red cell production, but rather the result of either increased red cell destruction (haemolytic anaemia) or acute blood loss (haemorrhage). By contrast a normal or reduced reticulocyte count in an anaemic patient suggests the anaemia is caused by either a deficiency of a nutrient (e.g. iron, vitamins B12 folate) required for red cell production or bone marrow disease that is limiting red cell production.
The reticulocyte count can be used to monitor effectiveness of some anaemia treatments. For example, prescription of iron tablets to a patient with iron deficiency anaemia is associated with a reticulocytosis (increase in reticulocyte count) that begins around five to seven days after the start of treatment. Reticulocytosis provides the first objective evidence that the iron is having the desired effect of increasing bone marrow production of red cells. Clearly absence of such a response suggests the patient is either not taking the tablets or was never iron deficient, and an alternative cause of anaemia should be considered.
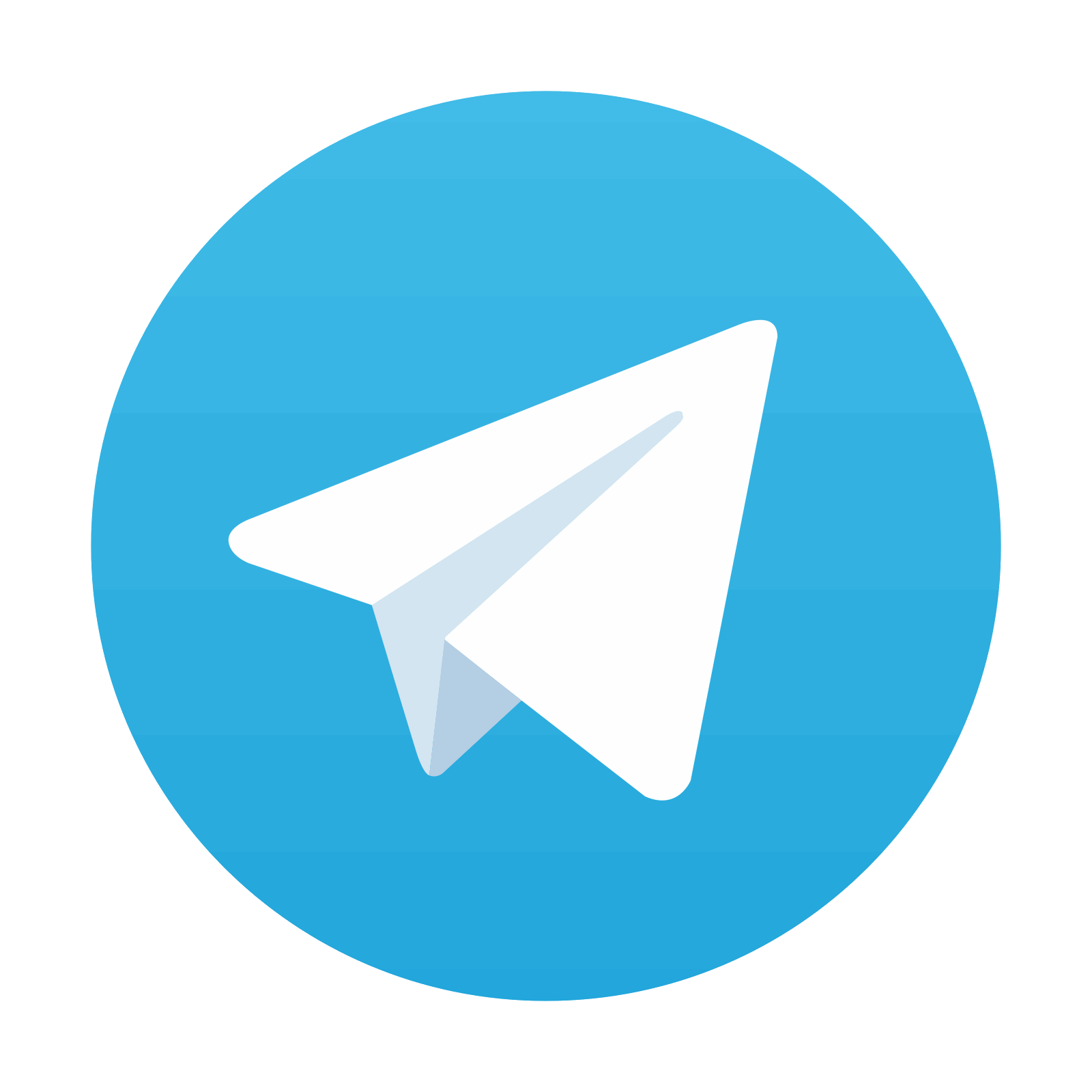
Stay updated, free articles. Join our Telegram channel

Full access? Get Clinical Tree
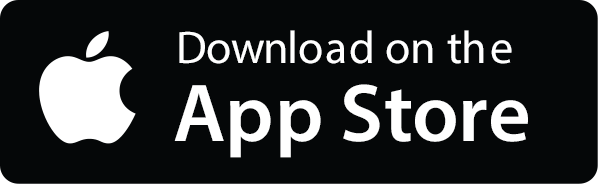
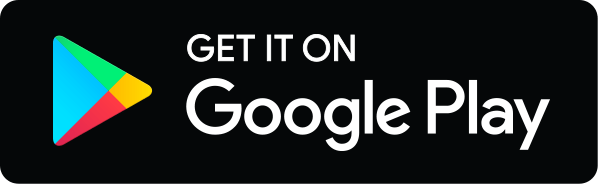