CHAPTER 10. Forensic Toxicology
Sarah Kerrigan and Bruce A. Goldberger
Toxicological analyses are undertaken in clinical and forensic laboratories to facilitate medical intervention, diagnosis, or treatment, and as part of the criminal justice system. Toxicology also plays a fundamental role in determining the cause and manner of death and is widely utilized in the workplace to deter drug use. Forensic comes from the Latin word forensis, which means “forum,” denoting a court or tribunal. Forensic toxicology is distinct from clinical toxicology in that it is often practiced within a legal domain for the purpose of upholding the law. However, clinicians and medical personnel do play an essential role in forensic toxicology. During medical treatment, they are often the first ones to make observations, diagnoses, and collect biological specimens from injured persons who may be the subject of a legal investigation. Analyses of specimens without the context in which they were collected are generally of limited value. To properly evaluate these tests, the patient’s or victim’s history of drug usage is needed. Furthermore, improperly collected specimens can yield improper or misleading results. Forensic nurses, because of their unique training, are in a position to aid clinicians, law enforcement officers, and legal personnel with investigations utilizing toxicological testing. They are familiar with the proper collection, storage, and testing of forensic specimens. In addition, they are experienced in conducting interviews and collecting histories, which will aid with the interpretation of the results from the analyses.
Subdisciplines of Forensic Toxicology
The three major subdisciplines of forensic toxicology are human performance toxicology, postmortem toxicology, and forensic urine drug testing. Human performance toxicology has to do with mental and physical effects of drugs that may, for example, impair judgment or coordination. Postmortem toxicology involves the investigation of drugs and poisons in circumstances of death. Forensic urine drug testing has become widespread in the military as well as in public and private sectors of the workplace.
Reasons for Drug Use
Unfortunately, in today’s society, the ingestion of a drug or drugs in the absence of medical supervision is commonplace. One common motive for the misuse of drugs includes the pursuit of euphoric or psychedelic effects. Many times these drugs are used to enhance social situations, used as a result of peer pressure, used out of curiosity, or used for “fun” or to “get high.”
Alternatively, drugs may be used to enhance mental, emotional, or physical well-being. For example, it is not uncommon for individuals to self-medicate for the relief of anxiety, depression, insomnia, or pain or to increase alertness or relaxation.
Toxicology Investigation
Toxicological findings may provide the necessary insight to explain behavioral or physiological effects (e.g., why a person drives off the road, attempts suicide, or unexpectedly falls unconscious). The use of prescription or over-the-counter (OTC) drugs in a manner inconsistent with accepted medical practice might be of relevance during a criminal investigation, particularly in a case involving driving a motor vehicle, operation of machinery, violent crime, or sexual assault.
As mentioned earlier, the selection, identification, collection, and preservation of biological evidence have a profound impact on the quality of analytical data and the interpretation of toxicological findings. To this end, the toxicologist relies on a variety of analytical techniques, of which some may provide results in minutes or hours, and others may require several days.
There is considerable overlap between the subdisciplines of forensic toxicology, which are largely governed by the natural laws of pharmacology and chemistry. Forensic toxicologists are commonly asked certain questions: Was a drug or chemical involved? If so, what was the drug or chemical? How much was taken and when? Was it responsible for the death or did it affect behavior? The key to answering these questions is an understanding of pharmacokinetics, pharmacodynamics, and analytical techniques.
Trends in Drug Use
The 2007 National Survey on Drug Use and Health (NSDUH), conducted by the Substance Abuse and Mental Health Services Administration (SAMHSA), indicated that an estimated 19.9 million Americans (8% of the population ≥ 12 years old) were illicit drug users, 57.8 million participated in binge drinking, and another 17 million were heavy drinkers. Drug abuse, whether it involves illicit substances or the misuse of therapeutics, is nondiscriminatory in that it has permeated almost every level of society to some degree. Trends in drug use among the young are largely governed by the perceived risk of drug use. As perceived risk decreases, use increases, and vice versa. These findings offer the hope that comprehensive drug prevention efforts, based on drug education and risk assessment, can curb drug use among young people.
In 2007, marijuana was the most commonly used illicit drug in the United States (10.4 million) followed by the nonmedical use of prescription drugs (6.9 million). Of these, an estimated 5.2 million used narcotic pain relievers, 1.8 million used tranquilizers, 1.1 million used stimulants, and 0.3 million used sedatives. In the same year, estimates for cocaine, hallucinogen, methamphetamine, and heroin users were 2.1 million, 1.0 million, 0.5 million, and 0.2 million, respectively (SAMHSA, 2007).
The Drug Abuse Warning Network (DAWN) estimates that in 2006, 1.7 million emergency department (ED) visits were associated with drug misuse or abuse. Of these, 31% involved an illicit drug, 28% involved a pharmaceutical drug, 7% involved alcohol use among minors, and the remainder involved combinations of illicit, pharmaceutical, and alcohol misuse. Over half of the drug-related ED admissions were attributed to illicit drugs, either alone or in combination with other substances. The most frequently reported drugs were cocaine (548,608), marijuana (290,563), heroin (189,780), and stimulants (107,575). Although no significant changes in drug-related ED visits were evident for the major illicit drugs between 2004 and 2006, visits related to the use of nonmedical or pharmaceuticals increased by as much as 38%. Benzodiazepine and opioid-related visits increased 36% and 43%, respectively. In 2006, an estimated 57,550 ED visits involved hydrocodone, 64,888 involved oxycodone, and 45,130 involved methadone.
Drugs in the military
A Department of Defense survey of substance abuse and health behaviors among military personnel showed that illicit drug use declined dramatically between 1980 (27.6%) and 2002 (3.4%). Substance abuse among military personnel has remained at about 3% since 1995, but it is significantly lower than rates of illicit drug use among the civilian population (5.8%). This downward trend seems to coincide with the 1990 guidelines proposed by the secretary of defense and the secretary of transportation to regulate and implement a comprehensive drug-testing program of military personnel. This deterrent against drug use appears to have been extremely successful and has become widespread in both the public and private sectors. Incentives for a drug-free workplace are improved safety and a reduction in financial losses caused by absenteeism, adverse health consequences, and accidents.
Drugs and crime
According to a 2002 study by the U.S. Department of Justice, as many as 29% of convicted inmates reported using illegal drugs at the time of the offense. The most common drugs were marijuana (10%), cocaine (11%), stimulants (5%), and heroin or opiates (4%). More than two thirds of jail inmates were found to be dependent on drugs or alcohol, and nearly 70% of probationers report past drug use. The most frequently reported drugs among probationers include marijuana (67%), cocaine (32%), stimulants (24%), hallucinogens (20%), depressants (16%), and heroin or opiates (9%). DWI offenders on probation parallel the overall probation population, which is cause for significant traffic safety concern; they report the use of marijuana (65%), cocaine (28%), stimulants (29%), hallucinogens (20%), depressants (15%), and heroin or opiates (6%).
Drugs and driving
According to the Fatality Analysis Reporting System of the National Highway and Transportation Safety Administration (NHTSA, 2002), 41% of the 38,309 fatal traffic crashes in 2002 were alcohol related. The exact number of traffic fatalities involving drugs other than alcohol is not known. However, the NHTSA estimates that drugs are used by approximately 10% to 22% of drivers involved in accidents, often in combination with alcohol. A study of fatally injured drivers from seven states showed that alcohol was present in more than 50% of the drivers, and other drugs were present in 18% of the drivers. An ongoing NHTSA study of nonfatally injured drivers has shown that compared to the general population, the percentage of young drivers (under 21 years old) who test positive for drugs has almost doubled. The incidence of positive drug findings in injured drivers who receive medical treatment ranges from less than 10% to as high as 40%. The incidence of drug-use among drivers arrested for motor vehicle offenses ranges between 15% and 50%. ED physicians may not have a legal obligation to report impaired drivers to the authorities, and as such, these drivers may not be held accountable for their actions.
In 2007, an estimated 9.9 million people reported driving under the influence of an illicit drug during the past year. This figure corresponds to 4% of the population aged 12 years or older. The highest rates of drug use while driving were reported among 18- to 21-year-olds (12.5%). In 2007, an estimated 31.4 million people in the United States (12.7%) reported driving under the influence of alcohol at least once during the previous year. Highest rates of alcohol-impaired driving were reported among 18- to 25-year-olds (22.8%), and males were nearly twice as likely as females to have driven under the influence of alcohol (SAMHSA, 2007).
Drugs and health
According to the 2007 National Survey on Drug Use and Health, of the 19.9 million Americans who were illicit drug users, an estimated 7.5 million were in need of substance abuse treatment. A further 19.3 million were in need of treatment for alcohol. However, only 1.3 and 1.6 million received treatment for drug- and alcohol-related problems. Dependence and suicide, followed by psychic effects, are the most frequently cited motives for drug use. Common reasons for drug-related admission to the ED are overdose, detoxification, unexpected reaction, chronic effects, or withdrawal. Unexpected reactions and overdoses were the predominant reasons for ED contact in episodes involving gammahydroxybutyrate (GHB), miscellaneous hallucinogens, phencyclidine (PCP), inhalants, methylenedioxymethamphetamine (MDMA, Ecstasy), amphetamines, ketamine, marijuana (THC), lysergic acid diethylamide (LSD), and alcohol in combination with other drugs. Detoxification is a common reason for ED contact for episodes involving heroin and cocaine.
Despite being perceived as a low-risk drug, marijuana is second only to cocaine in drug-related ED visits. Drug use remains somewhat regionalized, accounting for unrepresentative statistics in certain metropolitan areas and states. For example, LSD, GHB, and the designer amphetamines are more prevalent in California, Florida, and New York, where the drug-culture associated with the “rave” scene is popular.
Club drugs
“Club drugs,” which are becoming increasingly popular with young people, are commonly encountered at nightclubs and raves. These include the synthetic designer amphetamine MDMA, GHB, LSD, and methamphetamine, as well as illegally diverted and trafficked therapeutics, such as ketamine and Rohypnol (flunitrazepam). These drugs have been somewhat popularized by the rave culture and the false perception that they are not as harmful as mainstream illicit drugs such as cocaine and heroin. Statistics from DAWN show that ED mentions involving these drugs, notably MDMA and GHB, have increased at an alarming rate since the early 1990s (Fig. 10-1). Legislative efforts to curb this trend have included federal scheduling and inclusion of these drugs into the Controlled Substances Act. These legal efforts will be supported by a $54 million educational campaign, launched by the National Institute on Drug Abuse and partner organizations, to raise awareness of the dangers of GHB and other club drugs.
![]() |
Fig. 10-1 (From Drug Abuse Warning Network. (2003). DHHS Publication No. (SMA) 03-3780. Rockville, MD.) |
Pharmacokinetics
Pharmacokinetics describes the absorption, distribution, metabolism, and excretion of a drug. These factors influence the efficacy of the drug, its concentration at the active site, and the intensity and duration of the drug effect. Pharmacokinetic properties are used by pharmacologists, clinicians, and toxicologists to develop new therapeutics, understand the factors that govern use and abuse, determine how drugs can be detected in the body over time, and interpret their effects on human performance.
Routes of administration
Onset of action, duration of effects, intensity, and the “quality” of the drug experience may vary, depending on the route of administration. Intravenous (IV) drug administration provides maximum drug delivery and rapid onset of effects. The injection of a drug bypasses the body’s natural safeguards. Intravenous administration is complete and not affected by gastrointestinal absorption or intramuscular or subcutaneous absorption. In addition, IV drug administration also avoids the liver (first-pass metabolism) and is transported directly to the site of action without being inactivated by liver enzymes. Complications of intravenous drug use includes the transmission of infectious diseases such as human immunodeficiency virus (HIV) and hepatitis, as well as infection, emboli, blood vessel occlusion, thrombosis, and irritant effects (Box 10-1). For these reasons, inhalation and smoking are popular alternatives because these routes mimic IV administration. When a drug is smoked, it is rapidly absorbed in the lungs and transported to the brain via the arterial blood supply. Smoking is a preferred route of crack cocaine administration because of rapid onset, prolonged duration, intensity, and euphoria. However, pipes and smoking apparatus become hot and may burn the lips. The efficiency and speed of drug delivery increase the reinforcing effect of the drug and its abuse liability. However, not all abused drugs are amenable to all routes. The relative advantages and disadvantages of oral, inhalation, smoking, intravenous, intranasal, and dermal drug delivery are summarized in Table 10-1.
Box 10-1
HIV |
Viral hepatitis (B and C) |
Sexually transmitted infection (gonorrhea, syphilis) |
Bone and joint infection |
Abscesses and cellulitis |
Endocarditis |
Tuberculosis |
Pneumonia |
Septic pulmonary emboli |
Septic arthritis |
Septic thrombophlebitis |
Mycotic aneurysm |
Route | Advantages | Disadvantages | Drugs |
---|---|---|---|
Oral | Noninvasive | Slow absorption via GI tract First-pass metabolism Variable bioavailability Delayed onset Blunted reaction | Benzodiazepines, cannabinoids, GHB, LSD mescaline, opioids, peyote |
Inhalation | Noninvasive Rapid absorption | Limited to drugs with sufficient volatility | Solvents, gases, low boiling-point alkaloids |
Intravenous | “Rush” caused by bolus of drug Rapid onset | Invasive Deleterious health effects | Cocaine, methamphetamine, opioids |
Smoking | Rapid onset Rapid absorption Noninvasive | Pyrolysis of drug Not all drugs heat stable | Crack cocaine, marijuana, methamphetamine, opioids, PCP |
Intranasal | Noninvasive | Irritation of the nasal mucosa Variable absorption | Cocaine, methamphetamine, opioids |
Dermal | Noninvasive | Lipid-soluble drugs only Local irritation Delayed onset Blunted reaction, no “rush” | Fentanyl, nicotine |
Absorption
For a drug to exert a pharmacological effect, it must gain entry to the body by absorption and traverse biological membranes to its site of action. The route of entry determines the site of absorption: gastrointestinal, pulmonary, dermal, or parenteral. Unless the drug is administered intravenously, it is unlikely that all of it will be absorbed. The efficiency of this process, known as bioavailability, is the amount of drug that is absorbed relative to the amount that is administered. Physicochemical properties of the drug determine the efficiency of absorption, as well as the concentration of the drug, circulation, and surface area at the absorption site. Physical state, solubility, and formulation of the drug play important roles. Water-soluble liquids are absorbed more rapidly than oils, solids, or sustained-release formulations. Because absorption largely depends on diffusion, the greater the concentration gradient across the membrane (the higher the drug concentration), the faster the drug will be absorbed. A large surface area, such as the capillary-rich surface of the lungs or microvilli of the small intestine, facilitates absorption.
The pH and pK a of the drug determine how and where the drug gains entry to different compartments of the body. The pK a is the pH at which the drug is half ionized (Table 10-2). The majority of drugs can be classified as acidic, neutral, or basic. Phospholipid membranes within the body are selectively permeable, depending on size, charge, and lipid solubility of the drug. These membranes are effective barriers against passive diffusion of ionized drugs, which must undergo alternative methods of transport, such as filtration, phagocytosis, pinocytosis, and active or facilitated transport.
Drug Street Name(s) | Half-Life | Duration of Effect (h) | Protein Binding (%) | Vd (L/kg) | PK a | Unchanged in Urine (%) | Principal Metabolite(s) |
---|---|---|---|---|---|---|---|
Cocaine | 0.7-1.5 h | 1-2 | 92 | 2-3 | 8.7 | <10 | Benzoylecgonine, ecgonine methylester, norcocaine |
Blow, horn, nose candy, jelly beans (crack), rooster (crack), tornado (crack), moonrock (crack and heroin), wicky stick (crack, PCP, marijuana), snowball (cocaine and heroin) | |||||||
Fentanyl | 3-12 h | 0.5-1 | 79 | 3-8 | 8.4 | 1-5 | Norfentanyl, hydroxyfentanyl |
GHB | 0.3-1 h | 2-5 | 0 | 0.4 | — | <5 | — |
Goop, grievous bodily harm, good hormones at bedtime, max, soap | |||||||
Heroin | 2-6 m | 3-6 | 40 | 25 | 7.6 | <1 | 6-Acetylmorphine, morphine, glucuronide conjugates |
Smack, hell dust, big H, thunder, nose drops (liquefied heroin), crop (low-quality heroin), dragon rock (heroin and crack), A-bomb (marijuana and heroin) | |||||||
Ketamine | 3-4 h | 0.5-2 | 30 | 3-5 | 7.5 | 2-5 | Norketamine, dehydronorketamine, glucuronide conjugates |
Cat valium, K, jet, special K, super acid | |||||||
Lysergic acid diethylamide (LSD) | 3-4 h | 8-12 | — | 0.3 | 7.8 | <1 | NorLSD, 2-oxo-3-hydroxy-LSD |
Acid, acid cube, backbreaker, battery acid, doses, dots, Elvis, loony tunes, pane, superman, window pane, zen | |||||||
Marijuana (cannabinoids) | 1-3 d (naive) | 2-4 | 97 | 4-10 | 10.6 | <1 | 11-nor-Δ 9-carboxy-THC |
Bud, dope, ganja, herb, hydro, indo, Mary Jane, shake | 3-13 d (chronic) | ||||||
Methamphetamine | 6-15 h | 2-4 | 10-20 | 3-7 | 9.9 | 43 | p-Hydroxymethamphetamine, amphetamine, |
Biker’s coffee, chalk, chicken feed, crank, crystal meth, glass, go fast, ice, meth, poor man’s cocaine, shabu, speed, stove top, trash, yellow bam | p-Hydroxyamphetamine | ||||||
MDMA | 6-9 h | 2-4 | — | 5-8 | — | 65 | Methylenedioxyamphetamine |
Disco biscuit, ecstasy, hug drug, go, X, XTC | |||||||
Morphine | 1.3-6.7 h | 3-6 | 35 | 2-5 | 8.1 | <10 | Glucuronide conjugates, normorphine |
Phencyclidine (PCP) | 7-46 h | 2-4 | 65 | 5-8 | 8.5 | 30-50 | 4-Phenyl-4-piperidinocyclohexanol, 1-(1-phenylcyclohexyl)- 4-hydroxypiperidine |
Angel dust, busy bee, cadillac, elephant tranquilizer, embalming fluid, hog, jet fuel, killer weed, tick | |||||||
Phenobarbital | 2-6 d | 10-20 | 50 | 0.5-0.6 | 7.2 | 20-35 | p-Hydroxyphenobarbital, dihydrodiol, glucuronide conjugates |
Strong acids have low pK a’s (pH 1 through 5), which means that at physiological pH (7.2) they are mostly ionized. This state restricts the mobility of the drug because passive diffusion is not favored (Table 10-3). The pK a affects absorption and distribution of a drug. For example, the gastrointestinal tract varies widely in pH, from the buccal cavity (pH 7) to the stomach (pH 2), small intestine (pH 6), duodenum (pH 7), ileum and colon (pH 8). As a result, uncharged acidic drugs tend to absorb well in the stomach. Strong bases, however, which have high pK a values, are predominantly charged at physiological pH values. Even in the most alkaline regions of the gastrointestinal tract (pH 8), basic drugs like methamphetamine (pK a 9.9) are predominantly charged.
Percentage of Ionized Drug PH units from PK a | |||||
---|---|---|---|---|---|
Drug Type | −2 | −1 | PK a | +1 | +2 |
Acidic drugs | 1 | 9 | 50 | 91 | 99 |
(e.g., acetaminophen, ampicillin, barbiturates, non-steroidal anti-inflammatory drugs, phenytoin, probenecid, THC metabolites) | |||||
Neutral drugs | 0 | 0 | 0 | 0 | 0 |
(e.g., carbamazepine, glutethimide, meprobamate) | |||||
Basic drugs | 99 | 91 | 50 | 9 | 1 |
(e.g., antiarrhythmics, antidepressants, antihistamines, cocaine, narcotic analgesics, PCP, phenothiazines, sympathomimetic amines) |
The pK a of the drug not only determines the absorption, but it also determines where the drug is most likely to reside. For example, when drugs are distributed into the sweat, which is slightly acidic (pH 5), basic drugs like cocaine and methamphetamine become charged and are trapped in the fluid in a process known as “ion trapping.” This process becomes important during neonatal drug exposure, which will be discussed later.
Distribution
As soon as the drug is absorbed and enters the circulation, the distribution phase begins. Once more, depending on the physicochemical properties of the drug and the surrounding pH, passive diffusion, filtration, or transport mechanisms distribute the drug to surrounding tissues and organs. On entry into the circulation, plasma proteins may bind the drug. This bound state not only limits entry into the tissues, but it also prevents interaction with receptors necessary to produce a pharmacological reaction. Simultaneous administration of multiple, highly bound drugs can inadvertently increase the free concentration of drug, resulting in an acute toxic reaction. Extensively protein bound drugs may have a delayed onset or prolonged duration of action (see Table 10-2). Highly perfused tissues, such as the heart, liver, kidney, and brain, come into contact with the drug much faster than muscle and fat, which are perfused at much slower rates and take much longer to reach equilibrium with circulating drug.
Drugs that are lipid soluble are distributed more readily into the tissues and may penetrate the blood-brain barrier. Tetrahydrocannabinol (THC), the active constituent of marijuana, is lipophilic. It is distributed and stored in tissues and fat depots within the body, accounting for its gradual release and long half-life (see Table 10-2). The extent to which a drug is distributed in the body is given by the volume of distribution (Vd), which is a function of lipophilicity, protein binding, and pK a. Highly water-soluble (hydrophilic) drugs, such as ethanol (Vd 0.5 L/kg), are distributed mainly in the body water and have low volumes of distribution (Vd < 1 L/kg). Conversely, drugs with large volumes of distribution, for example, antidepressants (Vd 10–20 L/kg), are more likely to distribute throughout the body into the tissues. Heroin (Vd 25 L/kg) is capable of penetrating the protective endothelial and glial cells of the blood-brain barrier, whereas morphine (Vd 2–5 L/kg) does not.
METABOLISM
Most drugs are highly metabolized by the body with only relatively small amounts being excreted unchanged (see Table 10-2). To facilitate the process of elimination and to prevent the accumulation of drugs, several different types of biological transformations occur that increase the water solubility of the drugs. Metabolism can affect the pharmacological activity of the drug, sometimes eliminating activity (e.g., cocaine metabolism to benzoylecgonine) or increasing it (e.g., heroin metabolism to 6-acetylmorphine and morphine).
During phase I metabolism, enzymes, such as cytochrome P450, monoamine oxidase, cholinesterase, and others, oxidize, reduce, hydrolyze, and dealkylate drugs, rendering them more hydrophilic by introducing polar moieties. This process occurs throughout the body, particularly in the liver, but also in the kidneys, blood, lungs, skin, and gastrointestinal tract. During phase II metabolism, water-soluble conjugates are formed between polar functional groups on the drug and endogenous ligands such as glucuronic acid, sulfate, and glutathione. Drugs that undergo extensive conjugation, such as morphine, may require enzyme hydrolysis prior to analysis, which will be discussed later.
Sometimes a drug may undergo biotransformation before entering the circulation. Enzymes in the gastrointestinal tract can metabolize orally administered drugs, reducing their pharmacological activity and clinical utility. When the drug has been absorbed in the lower intestine, the portal circulation transports the drug to the liver, without it ever having reached the general circulation. This “first pass” effect accounts for the reduced bioavailability of some orally administered drugs.
A great many variables can affect drug metabolism, including age, sex, genetic polymorphisms, health, disease, and nutrition. The expanding field of pharmacogenomics is beginning to elucidate the role of genes on drug metabolism. For example, differences in the abundance of certain isoenzymes of cytochrome P450 account for differences in drug metabolism between ethnic populations. These factors are of scientific importance not only for the interpretation of drug findings but also for development of new therapeutic agents.
Elimination
Drugs and their metabolites are eliminated principally via the kidneys and liver, each of which is perfused with more than 1 L of blood every minute. Thus, the efficiency of drug elimination depends on hepatic and renal blood flow. During renal excretion of drugs, glomerular filtration, reabsorption, and secretion occur. The resulting ultrafiltrate accumulates in the bladder via the collecting tubules. The fraction of drug bound to plasma proteins and the glomerular filtration rate determine the amount of drug that enters the tubular lumen of the kidney. Charged species are less likely to permeate the tubular cells and reabsorb, a process that significantly delays elimination of drug. In this way, the rate of elimination is variable, depending on the pH of the urine. The elimination of acidic drugs is enhanced by alkalinization of the urine using sodium bicarbonate. Conversely, basic drugs may be eliminated more readily by acidification of the urine using ammonium chloride. As much as 76% of a methamphetamine dose may be excreted unchanged in acidic urine, compared with only 2% in alkaline urine.
Hepatocytes in the liver secrete bile, which is stored in the gallbladder and is later excreted via the intestines in the feces. Biliary excretion is favored by large polar substances, but when metabolism renders a drug more lipid soluble, the drug may undergo reabsorption, a process known as enterohepatic recirculation.
When a fixed amount of drug is eliminated per unit of time (e.g., ethanol), the elimination is said to be zero order. However, most drugs follow a first-order elimination process, whereby a constant fraction of drug is eliminated over time. Half-life (T½) is a measure of the rate of elimination. One half-life is the time it takes for the concentration of drug in the plasma to decrease by 50%. These values are dependent on volumes of distribution and clearance rates, which may vary with age, sex, disease state, or drug interactions. The lungs (for elimination of volatile substances) and even sweat, saliva, hair, nails, and breast milk are all relatively minor excretory pathways, but they may be of some forensic importance and will be discussed later.
Pharmacological Effects
The pharmacological effect of a drug is a result of the drug’s interaction at a given receptor site. The concentration of free drug circulating in the blood is in equilibrium with the concentration of drug at the receptor site. An increase in the concentration of free drug modulates the receptor response and enhances the pharmacological effect. The mechanism by which a drug modulates a receptor is described by pharmacodynamics. The link between the amount of drug and its effect over time is the basis for establishing therapeutic and toxic drug concentrations for clinical management and intervention. Such pharmacodynamic responses account for the pharmacological effect of a drug and are known as the dose-response relationship. However, pharmacological effect can be intrinsically dependent on the time passed since the dose was taken, rather than with the concentration of drug in the blood. A given concentration of drug may produce widely variable effects, depending on the time since administration. This phenomenon is referred to as hysteresis. For example, after consuming alcohol, a person tends to feel more excited and euphoric during the initial absorption phase than during the elimination phase, during which time the person may feel sedated and depressed. CNS stimulants such as methamphetamine follow a similar cycle. A concentration of 200 ng/mL methamphetamine in blood may coincide with euphoria, exhilaration, restlessness, and stimulation during the initial absorption phase. However, several hours later during the elimination phase, the same concentration of drug may coincide with confusion, depression, anxiety, dysphoria, and exhaustion.
The pharmacological effect experienced by the user may be apparent from vital signs or involuntary reflexes. For the purpose of determining impairment or acute or chronic toxicity, blood is the matrix of choice. The presence of the drug in the urine is only an indication of drug exposure, over a period of hours, days, or even weeks, rather than an indicator of impairment. With the exception of ethanol, there is no direct correlation between the concentration of a drug in the blood and the level of impairment for legal purposes. Any impairment by drugs is generally inferred by correlating the concentration of the drug with observations made by arresting officers or others involved with the impaired individual. Factors such as tolerance can have a profound effect on the pharmacodynamic response in an individual. A quantity of cocaine sufficient to produce a mild “buzz” in a chronic user could be acutely cardiotoxic in a naïve user, resulting in coma and death.
Drug recognition
Drugs may affect normal behavior by enhancing or impairing human performance (e.g., cognition or psychomotor ability), depending on the dose and pattern of drug use. Drug recognition is an important ability for clinicians, toxicologists, and law enforcement personnel. Recognition of abused substances, including illicit drugs and therapeutics, that may have been incorrectly administered or combined with other drugs, is a valuable skill.
Impairment may be observed and documented by either a trained clinician or a drug recognition expert (DRE). The DRE program was developed by the Los Angeles Police Department in the 1980s and is now used throughout the United States and overseas. For law enforcement purposes, a series of physiological and psychomotor tests are used to determine the class of drugs that is likely present: central nervous system (CNS) stimulants, CNS depressants, narcotic analgesics, hallucinogens, PCP, cannabis, or inhalants. Clinical characteristics such as blood pressure, pulse, respiration, body temperature, nystagmus, ocular convergence, pupil size, and pupillary reaction to light can be useful indicators of drug use (Table 10-4). Other observable effects, such as tremors, coordination, gait, muscle tone, perception, diaphoresis, emesis, lacrimation, and appearance of the conjunctivae, may also provide valuable insight (Table 10-5). Abstinence syndromes resulting from chronic drug use produce effects that vary considerably from those caused by acute drug intoxication. These effects may be impairing and, in some instances, can be life threatening (Table 10-6).
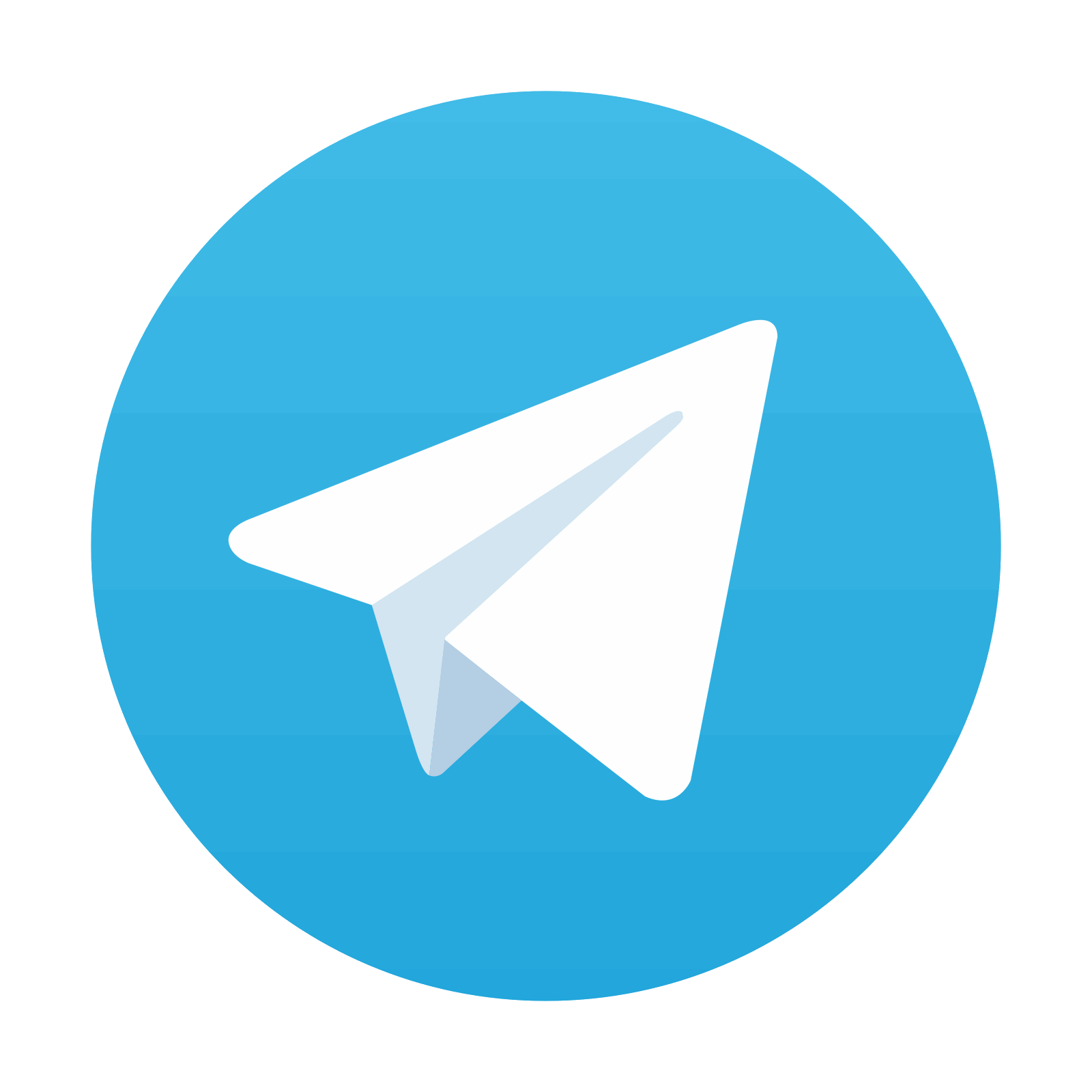
Stay updated, free articles. Join our Telegram channel

Full access? Get Clinical Tree
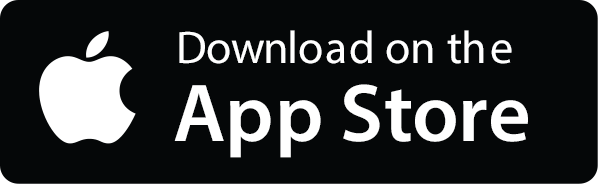
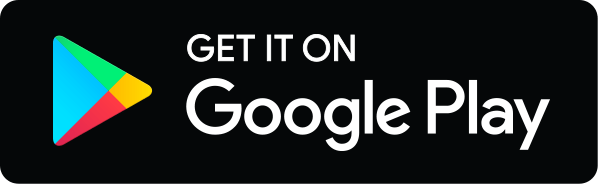
