Overview
The completion of the Human Genome Project (HGP) has changed how human health and disease is viewed. Physician assistants (PAs) practicing in this postgenomic age of medicine have an important role to play. First, this means PAs can take advantage of the genetic and genomic applications (tools) from the HGP research that are being streamed rapidly into clinical practice. These range from validated family medical history (FHx) tools, such as targeted interviews and web-based questionnaires to help PAs and other health care providers collect useful and accurate information and complete FHx data more quickly, to more widespread genetic and genomic testing, resulting from the dramatic cost reductions that have permitted inexpensive genomic sequencing. As a result, genetic testing and genomic sequencing are being harnessed across clinical specialties and in different clinical settings, including both inpatient and outpatient settings ( Table 11.1 ).
Clinical Specialty | Relevant Genetic or Genomic Diagnoses |
---|---|
Cardiology | Arrhythmia syndromes: atrial fibrillation, Brugada syndrome, long QT syndrome, short QT syndrome, CPVT Cardiomyopathies: arrhythmogenic right ventricular dysplasia, dilated cardiomyopathy, hypertrophic cardiomyopathy, left ventricular noncompaction cardiomyopathy, restrictive cardiomyopathy Coronary artery disease (and familial hypercholesterolemia) Familial aneurysms (thoracic) and aortopathies: syndromic and nonsyndromic—e.g., Marfan syndrome Congenital heart disease: isolated and syndromic Muscular dystrophies and heart disease |
Dermatology | Neurofibromatosis (type I) Tuberous sclerosis Familial melanoma Ehlers-Danlos syndrome Psoriasis Atopic dermatitis Albinism Vitiligo Alopecia areata |
Endocrine | Multiple endocrine neoplasia (MEN2 syndrome) Diabetes mellitus, type I Diabetes mellitus, type II |
ENT | Congenital hearing loss syndromes: Waardenburg, Ushers, and Alport syndromes Head and neck cancer (HPV, p53, nasopharyngeal carcinoma) Acoustic neuroma (NF II) Cleft lip and palate malformations Otosclerosis |
Gastroenterology | Inflammatory bowel disease: Crohn disease, ulcerative colitis Familial colorectal cancer syndromes: familial colorectal cancer, * FAP, Lynch syndrome Hereditary hemochromatosis Cystic fibrosis Wilson disease α 1 -Antitrypsin deficiency Celiac disease |
Gynecology | Familial breast and ovarian cancer syndromes: BRCA1, BRCA2, Lynch syndrome |
Hematology | Thalassemias: α and β Thrombophilias: factor V Leiden mutation, prothrombin mutation, MTHFR mutation, protein C or S deficiency, antithrombin III deficiency, dysfibrinogenemia Hemophilias: hemophilia A (classic hemophilia, factor VIII deficiency), hemophilia B (Christmas disease, factor IX deficiency) Sickle cell anemia Von Willebrand disease |
Musculoskeletal and rheumatology | Achondroplasia Spondyloarthropathies: ankylosing spondylitis Osteogenesis imperfecta Muscular dystrophies Ehlers-Danlos syndrome Marfan syndrome Spina bifida Congenital scoliosis Congenital hip dysplasia Clubfoot Osteoarthritis * Scoliosis * Osteoporosis * Rheumatoid arthritis * Systemic lupus erythematosus |
Nephrology and urology | Autosomal dominant polycystic kidney disease Wilms tumor IgA nephropathy End-stage renal disease * Hypertension * Prostate cancer * Bladder cancer * |
Neurology | Huntington disease Neurofibromatosis (type 2) Autism spectrum disorders (ASD) Alzheimer disease * Parkinson’s disease * Multiple sclerosis Essential tremor * Epilepsy * Dementia * |
Obstetrics | Prenatal genetic diagnosis: first- and second-trimester maternal serum screening, prenatal diagnosis of mendelian disorders and neural tube defects, population carrier screening, recurrent pregnancy loss |
Oncology | Refer to cancers related to specific organ system |
Ophthalmology | Retinoblastoma Congenital cataracts Congenital glaucoma Retinitis pigmentosa Age-related macular degeneration * Myopia (high myopia) Cataracts * Glaucoma * |
Psychiatry | Schizophrenia * Bipolar disorder * Unipolar major depression * Alcohol and substance abuse disorder * Anxiety disorder * Autism spectrum disorders * |
Pulmonology | α 1 -Antitrypsin deficiency Cystic fibrosis Asthma * Sarcoidosis * Lung cancer * |
* Denotes common disorders that often have a familial component and may be identified with collection and analysis of family history data.
Genetic and genomic applications can be used from diagnosis through treatment. For instance, FHx allows for the identification of patients who may benefit from genetic testing. Genetic testing can identify which patients have a disease. Once a patient is diagnosed, treatment may be determined by which genetic form of a disease a patient has. Finally, pharmacogenomics can determine which medication or dosage of medication from which an individual patient would benefit. These applications enable more and quicker individualized diagnoses and treatments for patients, with the long-term goal being to improve patient outcomes. This more individualized approach is part of the Precision Medicine Initiative. Precision medicine focuses on understanding individual variability in disease prevention, care, and treatment. Its aim is to understand how a person’s genetics, environment, and lifestyle can help to determine the best approach to prevent or treat conditions, disorders, and/or diseases (hereafter, these will all be referred to as disease) .
The main goal of this chapter is to provide an overview of some of the genetic and genomic applications and explain their relevance for clinical practice. It is assumed that readers will have familiarity with the fundamentals of genetics (e.g., DNA → RNA → protein, gene expression, Mendelian inheritance patterns).
Outcomes
- ■
Use genetic and genomic terminology with accuracy and precision.
- ■
Describe the genetic basis of human disease, including how human diseases are now being identified by their associated genetics and genomics;.
- ■
Define genetic heterogeneity.
- ■
Distinguish between variants, polymorphisms, and mutations.
- ■
Explain how variants, polymorphisms, and/or mutations influence human disease.
- ■
Distinguish between the types of genetic and/or genomic testing, including prenatal, newborn screening, carrier screening, presymptomatic testing, and diagnostic testing.
- ■
Elicit a correct family medical history (FHx) and accurately interpret pedigree data.
- ■
Identify relevant genetic resources (e.g., support groups, educational websites, research trials) for patients and families.
- ■
Provide genetic education for patients and families and make appropriate referrals for genetic counseling.
New role for physician assistants
Genetic disease is a term that likely conjures up images of rare syndromes and devastating illnesses. This is no longer an accurate view as research advances have shown that many common-occurring diseases like glaucoma, some forms of colon cancer, and premenopausal breast cancer have a genetic basis or a genetic component. In fact, a recent study used electronic health records (EHRs) to compare patient symptoms with the clinical features of 1204 Mendelian diseases. Patients who met a clinical criteria for one of the Mendelian diseases had genetic testing done for all of the Mendelian diseases, with 3.7% of patients (N = 21,701) found to have a genetic variant linked to one of the 1204 Mendelian diseases for which they received genetic testing. That is, 807 patients carried a pathologic variant for a disease such as cystic fibrosis (CF) or hereditary hemochromatosis.
PAs can begin to identify patients who are at increased risk for having a disease with a genetic component by obtaining and evaluating accurate family history (FHx) data. If indicated, the use of genetic testing may identify or diagnose patients. These diagnoses are valuable because they enable some patients to receive treatment before more expensive and invasive procedures are required. For example, patients with hypercholesterolemia might be identified and treated before they present with a myocardial infarction. This application of “genetic thinking” and use of genetic and genomic applications makes medical genetics the ultimate tool of PAs.
PAs, then, must do more than merely search for common diseases that have genetic components. They must incorporate “genetic thinking” into their daily clinical practice. This involves eliciting a targeted or detailed family history from each patient and analyzing it for Mendelian or complex patterns of disease. When a patient is identified as having or being at-risk for a disease with a genetic basis, predisposition or diagnostic genetic testing, medical monitoring, and treatment can be offered to reduce morbidity and mortality.
Identification of a patient who is a carrier or has a genetic disease will often lead the PA to other at-risk family members. They should be informed and referred to a medical provider for further evaluation. Even when genetic testing is not available, this information may still be valuable to at-risk family members because it may affect lifestyle and family planning decisions. Thus the diagnosis of a disease with a genetic component and access to genetic testing and/or genetic counseling is largely dependent on the well-informed PA.
Physician assistants as genetic gatekeepers
In a sense, PAs can act as genetic gatekeepers, opening the gate for patients to needed information and services, and at the same time closing it on inappropriate testing, as will be discussed later in this chapter. Patients can be offered a full range of associated services (for instance, genetic testing across the lifespan, prenatal testing, and predisposition diagnosis for adult-onset genetic disease). When indicated, PAs can provide genetic education or make referrals to genetic counselors and clinical geneticists.
Ultimately, acting as a genetic gatekeeper is not an effort to reduce the number of individuals who have a disease with a genetic component, nor should this ever be the goal. Rather, the aim is simply to offer the best possible treatment to at-risk patients and their family members to reduce associated morbidity and mortality.
Genetic testing
According to the National Library of Medicine’s Genetic Testing Registry, more than 10,000 disorders and diseases can be identified with genetic and genomic testing. This previously specialized testing is now part of clinical practice in primary care settings and across almost all specialties. PAs can now offer patients testing for relatively common diseases, such as CF and premenopausal breast cancer. Medical care and lifestyle choices can be made based on these test results. Couples planning to have children can avail themselves of universal carrier screenings to learn which diseases they may pass on to future children.
Treatment
Identification and testing of at-risk individuals is not necessarily useful unless treatment is available. Unfortunately, it is commonly believed, even among health care providers, that genetic diseases are not treatable. This is untrue; although most may not yet be curable, many are amenable to treatment. Moreover, treatment does not even necessarily mean medication; behavioral changes and lifestyle modifications can greatly reduce the morbidity and mortality associated with many of these diseases. An excellent example is CF. Better antibiotics, pancreatic enzymes, and aggressive medical management, as well as continuous health insurance have enabled those born with CF after 1990 to have a life expectancy of at least 40 years, an increase in survival of more than 20 years from 1970. Hemochromatosis can be treated successfully with dietary modification and therapeutic bloodletting. Additionally, there are some exciting treatments still in the laboratory that may one day result in cures, such as gene editing (in which clustered regularly interspaced short palindromic repeats [CRISPR], a group of repetitive DNA sequences, are used to delete or add DNA sequences).
Continuity of care
Once a patient has been identified as having a disease with a genetic component, it is essential that PAs work closely with specialists, who might provide future care. Furthermore, the PA should also become knowledgeable about the genetic aspects of the diagnosed disease to help the patient interpret and process the information they need to learn, such as an in-depth understanding of the disease, including the mode of inheritance.
The field of genetics is expanding rapidly. When new tests and treatments become available, patients who might benefit must be notified. Gradually, informing patients of the availability of genetic testing and treatment is becoming the standard of care.
Modern medicine’s language
Advances in genetics and genomics have changed the language of medicine. Daily discussions are now dotted with terms such as sequencing, polymorphism, or epigenetics. This vocabulary is arguably as important as terminology from anatomy and physiology, and PAs and advanced practice providers (APPs) should be fluent in the language of genetics and genomics. This fluency can empower PAs to better understand research and clinical literature, effectively recommend and order genetic and genomic tests, interpret laboratory reports, and intelligently discuss this specialized information with patients. , For example, the words allele and gene are often inaccurately used interchangeably. Humans are thought to have fewer than 17,000 genes, which are DNA segments that produce protein, but each gene can have multiple alternate forms. Alternate forms of genes are called alleles. So when referring to a variant of a gene, the correct word to use is allele. Similarly, the word genomic is now in routine use. Because we are in what has been described as “the postgenomic era,” what is the difference between genetic and genomic ? In general, genetic refers to a single or specific allele. In contrast, the term genome refers to the whole of an organism’s genetic information: a genome includes all the nuclear DNA, mitochondrial DNA (mtDNA), and ribonucleic acid (RNA) ( Box 11.1 ).
Allele: Different forms of a gene are called alleles . Alleles are variations in the DNA sequence of a gene. For example, A and B are specific alleles for the ABO blood group gene. Allelic variants can be conceptualized similarly to a type of biological maker, such as alpha-fetoprotein or prostate-specific antigen.
Familial clustering: When two or more biological family members have the same or a similar disorder, but there is no obvious Mendelian pattern of inheritance.
Genes: The fundamental unit of heredity, responsible for transmitting information from one generation to the next in gametes. The coding sequences of DNA are called genes . Genes are referred to as alleles (see Allele ).
Genome: The entirety of an organism’s genetic information. This means the coding sequences (i.e., genes) and the noncoding sequences—all the nuclear DNA, mtDNA, and RNA.
Genomics: Aims to understand the structure and functioning of different genomes (e.g., human primates, invertebrates), as well as the interplay of different genomes with different environments.
Heterozygous: The alleles at a genetic locus are different from one another. An individual with blood type AB is heterozygous at the ABO blood group locus.
Homozygous: The alleles at a genetic locus are identical. For instance, an individual with blood type O (i.e., genotype OO) is homozygous for the O allele.
Locus: Genes and their alleles are located on all chromosomes, and the position each one occupies is called a locus (plural, loci). For instance, the locus for the allele for β-hemoglobin (HBB) is on chromosome 11p15.5. HBB is a component (subunit) of a larger protein called hemoglobin, which is located on the inside of red blood cells.
Mitochondrial DNA (mtDNA): Mitochondria contain an independent circular genome with 37 alleles. Mitochondria are passed from mothers to both female and male children, and this is referred to as maternal inheritance .
Multifactorial inheritance: Any type of nonmendelian inheritance, including familial clustering; also referred to as complex inheritance .
Mutation: Accidental alterations or changes in our genetic material, DNA. There are three varieties of mutations: no effect, beneficial, or harmful. Most mutations are thought to have no effect on human health. These mutations generally occur in the noncoding region of our genome and are not believed to affect human health. These no effect mutations are sometimes called silent or neutral mutations. Mutations that occur in the coding region of our genome can be beneficial or harmful . Beneficial mutations confer health benefits; harmful mutations are usually associated with disease.
Polymorphism: A piece of DNA that has more than one form (allele), each of which occurs with at least 1% frequency, is said to be polymorphic ( poly , many; morph , forms). Polymorphisms are a normal part of genetic variability. Polymorphisms of the same gene may or may not have different functions.
Single nucleotide polymorphism (SNP; pronounced “snip”): A polymorphism that involves a change at a base pair of DNA (e.g., from a C to a G or an A to a T). SNPs are the most common type of genetic variation in humans. Some SNPs involve changes in the function of a protein that is made from a gene, but others are silent. Coding SNP (cSNP) is a SNP that occurs in a coding region.
Our shared inheritance I
Between any two humans, the variability at the genome level is only about 0.5%. , Greater variability is seen between members of other species; by comparing genomes, we have gained a greater understanding of the structure and function of different DNA sequences. Toward this end, hundreds of species have had their genomes sequenced. For instance, the Mexican axolotl’s (a salamander) genome was sequenced in 2018. Whereas the human genome is made up of about 3.2 billion base pairs, the Mexican axolotl’s genome contains up to 120 billion base pairs. This salamander is of particular interest because it is able to regrow its limbs even after amputation, and research into the genetic basis for this ability could one day help human patients. Please note the terms genome, genomes, and genomics will be used in relation to genomic sequencing or comparative genomics (see Box 11.1 ). Hereafter, the term genetic will stand for the phrase genetic and genomic testing.
Tour of the human genome
Every living organism has a genome. The human genome is composed of DNA (although some viruses have an RNA-based genome) and provides the genetic information for an organism’s development and maintenance. Of note, the human genome includes DNA from two different cell organelles, the nucleus and the mitochondria. DNA from the nucleus of the cell (nuclear DNA) and DNA from the mitochondria (mtDNA) together contain all the genetic information making up the human genome ( Fig. 11.1 ).

Anatomy of the human genome
In human anatomy, the term anatomic position is used as a reference for describing the relationship between different anatomic structures. Similarly, there are reference points that are used when discussing the human genome. For instance, although humans are diploid, the human genome is almost always discussed in terms of its haploid form and the HGP found that, in humans, the haploid form at the DNA level have the following characteristics:
- ■
Humans have ~3 billion base pairs.
- ■
Humans have fewer than 17,000 genes; these genes are arrayed on all 23 pairs of human chromosomes with more genes located on larger chromosomes genes. (Recall that genes are sequences of DNA that provide the instructions for building a protein). Genes are composed of two types of regions: (1) introns, which are not transcribed and (2) exons, which are transcribed.
- ■
Less than 1.5% of the human genome sequence contains areas, genes that code for proteins.
- ■
There is redundancy in the human genome. For instance, there are many gene families, meaning genes that code for related proteins (e.g., α-hemoglobin on chromosome 16, β-hemoglobin on chromosome 11). There are also older, nonworking genes within these gene families known as pseudogenes.
- ■
Approximately 50% of the human genome is made up of many repeating sequences of DNA; these repetitive elements are usually not part of the areas containing genes. Nevertheless, noncoding regions of the human genome do influence gene expression.
- ■
The human genome contains DNA from viruses.
Our shared inheritance II
Humans are more alike genetically than they are different. The HGP found that any two humans share an estimated 99.5% of their DNA in common. This finding reconfirms that Homo sapiens are all one species and all one race—the human race. , There is no genetic basis for separate races.
Genetic variation
Among humans, there is typically only 0.5% of variation in our DNA, which, on average, is 150,000,000 different base pairs. This genetic variation accounts for some of the differences observed in terms of skin color, hair texture, pharmacogenetic responses to medication (see the section on pharmacogenetics), and genetic susceptibility for disease. These and other differences may be because of changes—or variants —in the DNA sequence.
Variants, mutations, and polymorphisms
Changes in the DNA sequence are called variants. These are permanent changes in an individual’s DNA sequence. Every human being has variants. Variants occur in two ways: they are either inherited from a parent or are acquired throughout a person’s lifetime. New variants can result from the recombination portion of meiosis at conception or in response to environmental influences (e.g., chemical agents with mutagenic properties, radiation, and/or aging). ,
Variants can occur anywhere in the human genome, but those that occur within a gene and change the protein produced are called allelic variants. For each gene, there is usually one protein that is most common and is referred to as the wild-type allele. All other less common versions of the gene are referred to as variants or mutants. A related term, polymorphism, refers to variants, usually in more than 1% of the population, that can be benign or pathogenic.
When a sequence change within a gene is associated with disease, the terms used are pathologic variants or, previously, mutations ( Fig. 11.2 ). It was assumed that any individual with the mutant variant would show signs of disease. Nevertheless, further study has revealed that other individuals can carry the same mutation and do not currently show signs of the disease. In other words, in some individuals the so-call mutant variant was not pathologic.

In 2015, the American College of Medical Genetics acknowledged that the terms mutation and polymorphism are often confusing and therefore recommended that the term variant be used instead, along with one of these descriptors: (1) pathogenic, (2) likely pathogenic, (3) uncertain significance, (4) likely benign, or (5) benign. This is important to know because the laboratory reports PAs review will most likely incorporate this new nomenclature. So, in order to comprehend the laboratory test, PAs need to use this terminology.
New variants arise with regularity in the genomes of all living organisms, including humans. The variant rate in humans, or how frequently variants occur, can be calculated or estimated for both nuclear DNA (DNA) and mitochondrial DNA (mtDNA). Each of us inherit variants from our biological parents and, on top of that, we each have new variants that arise in us before birth. It is estimated that the average individual possesses more than 200 variants (per diploid genome).
With the understanding that genetic disease can never be eliminated because new genetic variants arise in the human genome at a somewhat regular rate, it is unlikely that genetic disease can be eradicated, and this is not the goal of genomic medicine. Instead, the focus is on the identification, diagnosis, and treatment of individuals who carry a variant associated with disease susceptibility or who already have a disease associated with a variant or variants. Identification of individuals who are at-risk allows treatment to delay the onset of disease or at least allow for improved monitoring for disease onset. Also, the earlier diagnosis of someone with a variant-specific disease enables earlier mitigation and, hopefully, reduction in the associated morbidity and mortality.
A genetic and genomic view of human disease
Almost every human disease likely has a genetic component. Most diseases have a weak genetic component, meaning an individual may be vulnerable (susceptible) to developing a disease. Other diseases have a strong genetic component, meaning an individual has a higher likelihood of developing a disease. The relative strength or magnitude of a genetic effect for a disease can be categorized along a continuum ranging from susceptibility (multifactorial disorders) to causative (Mendelian) alleles. , Although we typically consider two broad genetic classes of human disease (multifactorial and Mendelian), it may be more appropriate to consider the spectrum of the genetic component of human disease ( Fig. 11.3 ).

Mendelian diseases are not rare, but they occur less frequently than complex genetic diseases. Examples of Mendelian diseases include hypercholesteremia, CF, and sickle cell disease (SCD). In these diseases, a mutation in one or more alleles is directly associated with the disease, so the term causative allele is used. Mendelian diseases typically exhibit a distinct pattern of inheritance (e.g., autosomal dominant, autosomal recessive, X-linked).
Complex diseases are common, accounting for most health problems in the general population. Some examples are venous thrombosis, asthma, familial hypercholesterolemia, and osteoarthritis. , These diseases are related with susceptibility alleles and/or environmental factors. Susceptibility means an individual is vulnerable or likely to develop a disease. A susceptibility allele confers an increased risk that an individual may develop it, but the susceptibility allele is not sufficient to cause the disease. Complex diseases usually do not show a recognizable Mendelian inheritance pattern (i.e., autosomal recessive, autosomal dominant, or X-linked) on a family pedigree. Nevertheless, every so often two or more family members have the same or similar complex disease, but there is not a recognizable Mendelian inheritance pattern. In this case, the disease “runs in the family;” this phenomenon is termed familial clustering or familial aggregation ( Fig. 11.4 ).

Molecular genetic characterization of human disease
Human diseases are now classified according to their molecular genetic characteristics. For example, clinicians will discuss breast cancer in terms of whether it is BRCA1 or BRCA2, which are the genes associated with many forms of breast cancer. We are learning more about the mechanisms of health and disease from the study of genes that are protective and deleterious. New categories of diseases have emerged, revealing that some diseases once thought to be a single entity are actually multiple distinct diseases that share a similar or identical phenotype, such as in the case of dementias or breast cancer. , For instance, there are different Mendelian or complex subtypes of breast cancer, Alzheimer disease (AD), and Parkinson disease. When there are genetically distinct subtypes of what clinically appears to be the same disease, this phenomenon is known as genetic heterogeneity.
These different forms of disease are clinically indistinguishable from each other but can be differentiated with specific diagnostic genetic testing. Genetic testing laboratories offer a panel genetic testing (also known as a multiple-gene panel) to distinguish forms of disease. Panel genetic testing involves next-generation sequencing (NGS) technology to simultaneously sequence multiple genes or pathologic variants within those genes. The genes or pathologic variants are chosen a priori by committees of professionals with expertise in studying or treating diseases that appear to be the same disease but are in fact characterized by genetic heterogeneity. This testing allows for the timely diagnosis of individuals. This identification allows for medical monitoring or evaluation, patient education about lifestyle, and prevention practices, all with the goal of reducing disease morbidity and mortality.
Panel genetic testing is employed for many diseases (phenotypes) with known genetic heterogeneity, such as breast cancer, AD, and Parkinson disease, or even to diagnose patients with thrombotic events who do not have evidence for an acquired coagulopathy but do have a FHx suggestive of an inherited thrombophilia. Likewise, for individuals with a clinical presentation of breast cancer, genetic panel testing can identify and characterize any pathologic variants in the known breast genes (e.g., BRCA1, BRCA2). Test results may inform treatment and provide prognostic information.
Again, when there are genetically distinct subtypes of what clinically appear to be the same disease, this is known as genetic heterogeneity.
Now that diseases are being classified and treated according to their molecular genetic origin, disease names like AD, Parkinson disease, or breast cancer are no longer considered unique disease entities. Instead, the diagnosis of a disease like AD is more like a phenotypic description than a precise diagnosis. On a practical level, phenotypic-description-diagnoses are and will be acceptable in many clinical settings; however, more precise diagnoses may be necessary, especially when treatment decisions vary or depend on the underlying molecular genetic diagnoses.
Going forward, genetic heterogeneity is a term with which PAs will have to become very familiar. This is because scientists have evidence that complex human diseases are likely the result of many, many individual or family variants, sometimes called private variants (or private mutations ). Evolutionary forces generate a lot of genetic heterogeneity over time and, of course, new variants are introduced into each generation and each new human. With the advent of inexpensive NGS, it is likely that many of these pathologic variants will be identified and cataloged for further study.
Taken all together, this information may seem daunting, but PAs can best serve their patients during this postgenomic era by doing something they excel at: taking a careful history and physical. Here we review the basic tenets of eliciting and interpreting FHx data.
Family medical history
Although FHx has always been a standard part of data collected from patients, greater emphasis is now placed on FHx data. Typically, patients self-report their FHx. This information should be collected in a systematic fashion via a patient interview or standardized questionnaire or a combination of both methods.
Collecting family medical history data
FHx is limited to the personal medical histories of individuals to whom one is biologically related. The gold standard is a three-generation FHx constructed using standard pedigree symbols. Clinical practice guidelines endorse a comprehensive FHx, including multiple generations, usually three generations, drawn in a pedigree format. Standardized pedigree symbols should be used. Medical information relevant for the current clinical practice setting should be gathered. For a three-generation pedigree, FHx information should be gathered and listed about each of a patient’s closely related relatives ( Table 11.2 ) (i.e., first-degree relatives, such as parents, siblings, and children; second-degree relatives, such as grandparents, aunts, and uncles; and third-degree relatives, such as cousins and great-grandparents). Pertinent information includes the following: (1) diagnoses, (2) age at onset (AAO) or age at diagnosis (AADX) of any diseases, and (3) age at and cause of death ( Tables 11.3 and 11.4 ).
Degree of Relatedness | Definition and Relatives |
---|---|
First-degree relative |
|
Second-degree relative |
|
Third-degree relative |
|
Name |
Age or year of birth (date of birth is preferable, if known) |
Age at death and cause of death |
Ethnic background of each grandparent |
Relevant health information (see Table 11.4 ) |
Relevant symptoms or diagnoses and age at diagnosis (if known) |
Information regarding pregnancies, including infertility, spontaneous abortions, stillbirths, and pregnancy complications |
Developmental delay and learning disabilities |
Dysmorphic features or congenital anomalies |
Consanguinity issues |
Date and write your name legibly on the pedigree together with an explanation of any abbreviations |
Alcohol abuse | Drug abuse |
Allergies | Emphysema |
Alzheimer disease or dementia | Epilepsy or seizures |
Anemia | Glaucoma |
Asthma | Hearing loss |
Arthritis | Heart trouble |
Birth defects or malformations | Hemochromatosis or “iron overload” |
Any cancer | High blood pressure |
Breast cancer | Infertility |
Ovarian cancer | Kidney trouble (renal disease) |
Uterine cancer | Memory loss or Alzheimer disease |
Lung cancer | Mental illness |
Colon or rectal cancer | Mental retardation |
Prostate cancer | Multiple miscarriages |
Thyroid cancer | Neurofibromatosis |
Brain cancer | Obesity |
Melanoma | Osteoporosis or “hip fracture” |
Other cancer | Phenylketonuria or “metabolic” disease at birth |
High cholesterol | Sickle cell anemia |
Chronic infections | Smoking |
Clotting or bleeding problems | Stillborn or infant death |
Depression | Stroke |
Diabetes mellitus | Violence or domestic abuse |
Down syndrome | Other: ________________ |
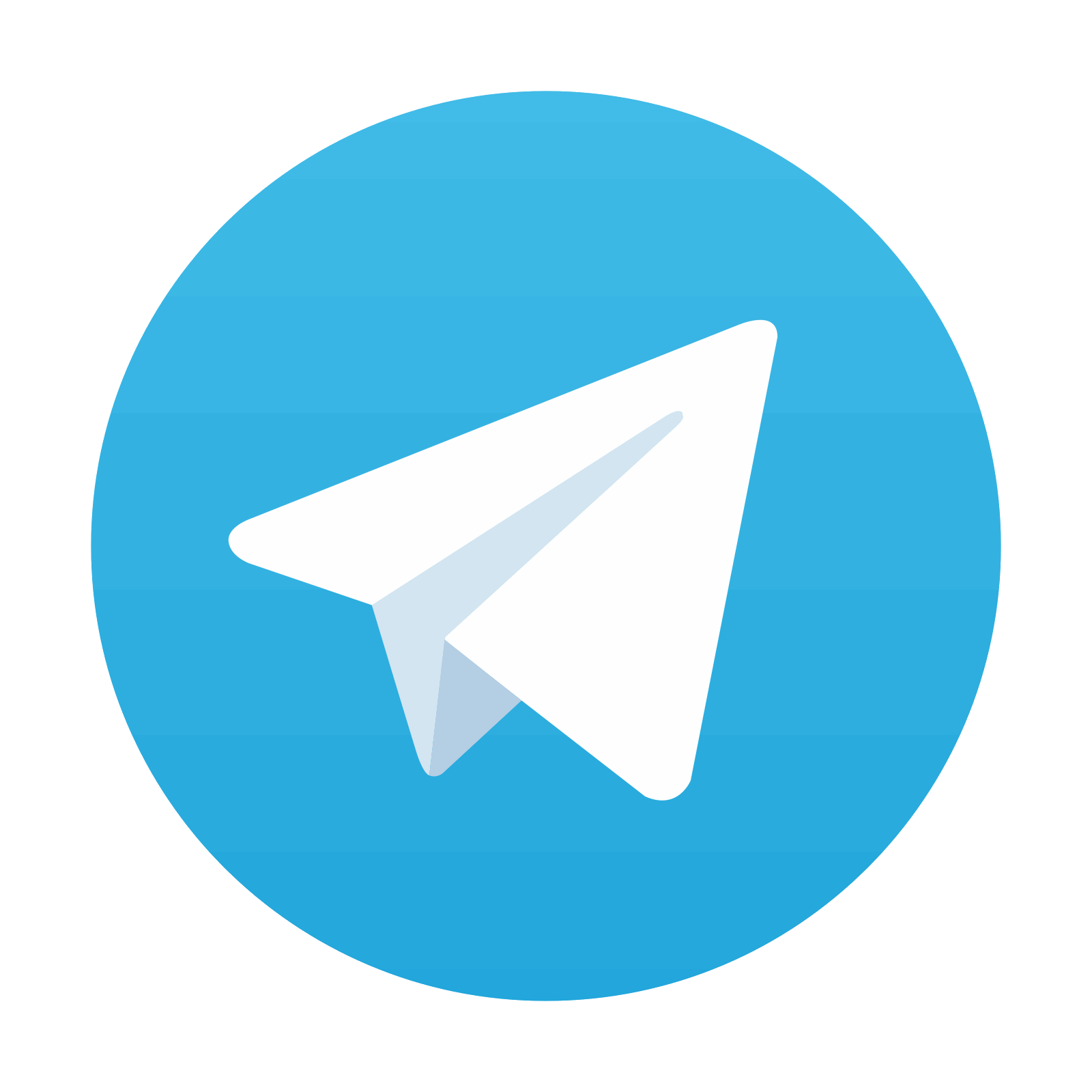
Stay updated, free articles. Join our Telegram channel

Full access? Get Clinical Tree
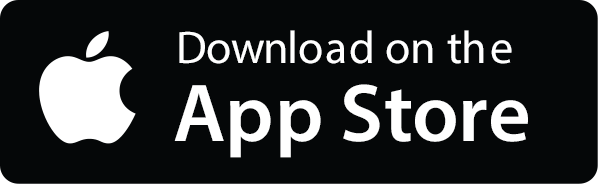
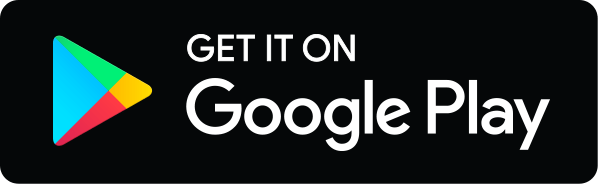
