Sleep
Kathy P. Parker
Rebecca A. Gary
Sandra B. Dunbar
▪ INTRODUCTION
Physiological changes that accompany normal sleep may have adverse effects on patients with cardiovascular disease, and because cardiac patients as a group also have a high prevalence of sleep abnormalities, attention to sleep in overall cardiovascular care has become increasingly important.1,2 Cardiovascular nurses are well positioned to assess sleep patterns, identify poor sleep quality and quantity, intervene to prevent sleep loss, educate and counsel cardiovascular patients regarding sleep, and work with the interdisciplinary team to assure treatments for sleep and sleep-related problems. To assist nurses in helping patients with cardiovascular disease achieve adequate, restful, and restorative sleep, this chapter reviews normal sleep and sleepiness, changes in cardiopulmonary and other system functions during sleep, sleep problems commonly seen in patients with cardiovascular disease, and appropriate management.
▪ NORMAL SLEEP
Sleep and Sleepiness
The human need for sleep has been recognized throughout the centuries, and few physiological phenomena have received as much attention from scholars, scientists, poets, and other literary figures. Before the twentieth century, sleep was thought to be a simple, passive phenomenon—a state often described as existing between waking and death.3 Although much remains to be fully understood about the topic, the modern study of sleep has revealed some of its secrets. Sleep is now understood as an active process regulated by a multiplicity of behavioral, neuroendocrine, and central nervous system factors.4,5 Insufficient and/or poor quality nocturnal sleep and daytime sleepiness adversely affect important clinical outcomes.6, 7, 8, 9 Numerous primary sleep disorders10 have been recognized, and the field of sleep medicine is now a bona fide, empirically based subspecialty.11
The modern definition of sleep is “a reversible behavioral state of perceptual disengagement from and unresponsiveness to the environment.”4 Sleep is further defined according to behavioral and physiological criteria. Behavioral criteria include quiescence, closed eyes, decreased response to external stimuli, recumbent position, and reversible unconsciousness.4 Physiological criteria are based on recordings from a polysomnogram that includes electroencephalography (EEG), electro-oculography (EOG), and electromyography (EMG)12 (Figs. 8-1 and 8-2).
Daytime sleepiness refers to the tendency or propensity to fall asleep during the day. In normal individuals, sleepiness typically has a biphasic circadian rhythm,13 with an increased sleep tendency in the mid-afternoon and, as is well known to nightshift workers, in the early morning hours13,14 (Fig. 8-3). In fact, a continuum between being very alert and very sleepy (often referred to as arousal state) provides a background for all waking endeavors and is a far more important dimension of human function than commonly recognized. Many adults are chronically sleepy in the daytime because of insufficient or disrupted night-time sleep. The problem may initially go unnoticed when masked by stimulating factors such as movement, excitement, high motivation, or hunger. However, daytime sleepiness can be unmasked by situational factors such as boredom, a warm dark room, or a prolonged dull task.13 Although poor nocturnal sleep can cause sleepiness, abnormal daytime sleep can also adversely affect nocturnal sleep. Thus, a complete assessment includes an examination of nocturnal and daytime sleep/wake patterns.
Stages of Sleep
Typical EEG patterns during wakefulness and sleep are shown in Figure 8-2. During relaxed wakefulness with the eyes open, the EEG consists predominantly of mixed frequency (cycle per second; Hz), low-voltage activity (low amplitude), or desynchronized brain-wave activity. Rapid eye movements (REMs) and blinks may occur, and muscle tone is usually at its highest level. With eyes closed, alpha waves are often noted (8 to 12 Hz).15
Sleep onset is heralded by a general slowing of the EEG activity and the emergence of delta waves (4 to 7 Hz) during more than 50% of the epoch. Sleep then progresses through several stages of nonrapid eye movement (NREM) and rapid eye movement (REM) sleep and cycles (an NREM/REM cycle) that are well described and form characteristic patterns in individuals and groups. NREM sleep is divided somewhat arbitrarily into three stages based on the EEG pattern. Sleep depth increases from stage 1 to stage 2 to slow wave sleep (SWS; deep sleep; previously defined as stages 3 and 4) based on the fact that the sleeper becomes more difficult to awaken.
In stages 1 and 2, or light sleep, the EEG consists of relatively low-amplitude waves with a predominant frequency of 2 to 7 Hz. High, narrow, vertex, sharp waves may appear late in stage 1. Stage 2 is identified by two sporadic waveforms that stand out from the background EEG: sleep spindles and K complexes. Sleep spindles are waxing-waning bursts of waves in the 12 to 14 Hz range.4,12 They originate in the thalamus and are thought to reflect impulses that inhibit the relay of sensory information to the cerebral cortex.16 K complexes consist of a sharp negative wave (upward deflection by EEG) followed by a slower positive wave (downward deflection).17 They occur spontaneously and in response to mild external stimuli, such as sounds. SWS is differentiated by the percentage of slow (0.5 to 2 Hz), high-amplitude (>75 μV) EEG waves (referred to as synchronized brain-wave activity). They account for 20% to 50% of the waves in each
epoch. The eyes are relatively quiet during NREM sleep, except for slow rolling movements that usually occur at the beginning of stage 1 and disappear in stage 2. Muscle tone is moderately reduced from the waking level.12,15
epoch. The eyes are relatively quiet during NREM sleep, except for slow rolling movements that usually occur at the beginning of stage 1 and disappear in stage 2. Muscle tone is moderately reduced from the waking level.12,15
There are two types of REM sleep. Similar to waking, tonic REM sleep is characterized by desynchronized brain activity—a mixed frequency, relatively low-amplitude EEG. However, in REM sleep there is also a complete loss of postural muscle tone caused by hyperpolarization of brainstem and spinal motorneurons.18 The sleeper has an active brain in a paralyzed body, with only the diaphragm and extraocular muscles retaining substantial tone. Some suggest that the purpose of this physiological phenomenon is to prevent the enactment of dreams. REM behavior disorder, in which there is loss of this normally occurring paralysis,
is typified by abnormal movements, behaviors, and dream enactment during REM sleep. Phasic REM sleep occurs intermittently and is characterized by bursts of REMs (for which the stage is named), muscle twitches in the face and distal extremities (potent motor excitation briefly overrides the paralysis), and fluctuations in blood pressure, heart rate, and breathing.18
is typified by abnormal movements, behaviors, and dream enactment during REM sleep. Phasic REM sleep occurs intermittently and is characterized by bursts of REMs (for which the stage is named), muscle twitches in the face and distal extremities (potent motor excitation briefly overrides the paralysis), and fluctuations in blood pressure, heart rate, and breathing.18
Approximately 80% of people awakened from REM sleep and 40% awakened from NREM sleep report having dreams. In NREM sleep, the mental activity tends to have a dull, sketchy quality without much basis in reality. In contrast, dreams recalled from REM sleep are usually vivid, well-formed, story-like narratives. Dreams include more visual imagery and emotional tone as the night progresses in relation to longer REM periods and greater intensity of phasic events.4 Penile and clitoral erections also often occur during REM sleep.
Sleep Cycles
Most people have their major sleep period at night, organized in a rhythmic sequence of sleep stages (Fig. 8-4). After a short period of relaxed wakefulness, a young adult enters stage 1 sleep, followed by a descent into stage 2 for 10 to 25 minutes and approximately 20 to 40 minutes of SWS. The sleeper then goes through stage 2 and has a brief REM period approximately 90 minutes after sleep onset (the period from sleep onset to the first REM period is referred to as REM latency). The cycle begins again and repeats another four to six times during the night. Slow-wave sleep occupies less of the second cycle and may then disappear, whereas REM periods lengthen across the night. Therefore, most SWS occurs in the first third of the night, and most REM sleep occurs in the last third. If an awakening occurs, the sleep cycle typically starts again with stage 1 sleep. Frequent disruptions of sleep prevent the normal progression into SWS and REM sleep and increase stages 1 and 2 sleep.4,15
Adults typically change their body position 40 to 50 times during a normal sleep period; the characteristics and number of movements that occur are relatively stable personal traits.19, 20, 21 Major body shifts often occur at changes from SWS to lighter NREM stages or from REM to NREM sleep. A sudden muscle contraction involving all or part of the body (hypnic jerk, hypnic myoclonus, or sleep start) often accompanied by intense visual imagery occasionally occurs at sleep onset and is normal; however, the frequency of the events may increase with stress or irregular sleep schedules.4,22
The Function of Sleep
The function of sleep remains a topic surrounded by controversy. Some have postulated that it is important for mental and physical restoration23,24 and energy conservation.25,26 Others propose that the primary function of sleep is the maintenance of synaptic and neuronal network function, information processing, and synaptic plasticity.27, 28, 29, 30, 31 Sleep deprivation studies have shown that total and
partial sleep loss impair well being and functioning, with mood being the most strongly affected, followed by cognitive and motor performance.32, 33, 34 Most agree that although the exact function of sleep remains to be discovered, it fulfils a vital need, one that is essential to human health and well being.35
partial sleep loss impair well being and functioning, with mood being the most strongly affected, followed by cognitive and motor performance.32, 33, 34 Most agree that although the exact function of sleep remains to be discovered, it fulfils a vital need, one that is essential to human health and well being.35
Sleep plays an important role in thermoregulatory36, 37, 38 and immune processes.39,40 Special areas in the hypothalamus and basal forebrain integrate temperature and sleep control through a network of complex interactive processes. For example, an increase in brain temperature before sleep onset increases sleep depth while deep sleep increases heat loss by stimulating vasodilatation and reduction of the metabolic rate. Peripheral signals coming from skin thermosensors going to these brain regions can also have a significant effect on sleep/wake state.37,41 In fact, vasodilatation of blood vessels in the feet in response to local warmth was recently shown to be an independent predictor of sleep onset.42,43 Many immune factors such as interleukin-1, interleukin-2, and tumor necrosis factor-α have been shown to promote deep sleep, possibly because of the associated increased heat production.40 Thus, the interaction of sleep, thermoregulation, and immunological responses may explain why patients become sleepy when having fevers and infections. Sleep deprivation has also been associated with reduction in the activity of natural killer cells in response to a bacterial or viral load, suggesting a direct link between sleep and immune function.39,44
Regulation of Normal Sleep
According to the Two-Process Model of Sleep Regulation,45 the major mechanisms controlling sleep and waking across time are: (a) a homeostatic process determined by previous sleep and waking; and (b) a circadian process that designates periods of high and low sleep propensity. The homeostatic process reflects the physiological need for sleep, which builds across the day and dissipates throughout the night (Fig. 8-5).10 A key indicator of this process is EEG slow wave activity, which is high during the beginning of a sleep episode but decreases as the night progresses. The circadian process, a sinusoidal rhythm of approximately 24 hours, is controlled by a biologic oscillator (suprachiasmatic nucleus). This process regulated sleep propensity and its effects a least in the early morning hours. The rhythm of core body temperature is a key indicator of the circadian process. The timing and duration of sleep are determined by the combined action of homeostatic and circadian processes via their influence on thermoregulatory and neuronal/neurohormonal systems.45, 46, 47 Factors that either oppose or enhance these processes can have significant effects on the timing, duration, and structure of sleep as well as daytime alertness.
Developmental Variations in Sleep Patterns
One of the most important factors affecting the pattern of sleep across the night is age (see Fig. 8-4). During the first years of life, the transition from wake to sleep typically occurs through REM sleep observed as active sleep in newborns when phasic muscle activity and eye movements can be observed. This is in sharp contrast to adults in whom sleep is normally entered through NREM sleep. The sleep cycle of a newborn occurs every 50 to 60 minutes compared to 90 minutes in the adult, and sleep is intermittently dispersed across both the day and night. Gradually, over a period of 2 to 6 months, infants develop a consolidated nocturnal sleep period once appropriate brain structures and process have developed.
SWS is at its peak in young children and is much deeper than that of adults. For example, it is not uncommon for a child’s clothes to be changed and to be put to bed without awakening. However, a subsequent decrease in SWS occurs across adolescence, a trend that continues to occur with age. REM sleep, as a percentage of total sleep time, is relatively well maintained across the entire life span.4
With increasing age, particularly in men, sleep becomes lighter and more fragmented (see Fig. 8-4). In contrast to young adults, older people usually spend more time in bed but less time asleep (reduced sleep efficiency) and are more easily awakened from sleep. The time needed to fall asleep (sleep latency) shows little change with aging, but more night-time awakenings, brief arousals, and stage changes occur.48 There is a striking reduction in SWS and an increase in stage 1 sleep, with little change in the percentages of stage 2 and REM sleep.4 Bedtime and wake-up time come earlier (circadian phase advance), daytime sleep tendency may be increased, daytime napping is more common, and tolerance for changes in the sleep-wake schedule is reduced. Sleep apnea (discussed later) and periodic leg movements (involuntary repetitive jerks) are more common in older adults and can contribute to sleep disruption.49, 50, 51, 52, 53, 54 Other factors may include poor sleep habits, a reduced activity level, psychological concerns, physical illness, and medications.48 Not surprisingly, older people often are dissatisfied with their sleep, complain of taking longer to fall asleep, and have more frequent night-time awakenings—all of which result in an increased using of sleeping medications.55,56 Insomnia has been associated with increased mortality in the elderly.57 However, it now appears that sleep duration, whether it be excessively short or long, may not be a mortality risk factor in this population but rather a function of the measurement of sleep close to the time of death and the number of concurrent medical conditions.58
▪ SLEEP PHYSIOLOGY
The physiological basis of nursing care has rested almost entirely on studies of responses during wakefulness. However, NREM sleep, REM sleep, and wakefulness are very different physiological states associated with state-dependent changes in the function of
most body systems.5 In healthy individuals, these changes are well tolerated; but in patients with cardiovascular disease, the sleep state may make them vulnerable to serious, sometimes fatal, complications.59
most body systems.5 In healthy individuals, these changes are well tolerated; but in patients with cardiovascular disease, the sleep state may make them vulnerable to serious, sometimes fatal, complications.59
Cardiovascular Function in Sleep
Cardiovascular control during sleep is primarily determined by variation in autonomic nervous system activity, which causes changes in blood pressure and heart rate, the major determinants of myocardial O2 demand.59,60 Increased parasympathetic tone and, to a lesser extent, decreased sympathetic tone, lead to a reduction in heart rate and cardiac output in NREM sleep. Vasodilatation causes a reduction in systemic vascular resistance and a 5% to 15% decrease in blood pressure which can impair blood flow through stenotic coronary blood vessels and trigger myocardial ischemia or infarction.59 In contrast, pulmonary artery pressure increases slightly.61,62 Baroreceptor gain is heightened and contributes to the reduction and stability of blood pressure. Brief surges in blood pressure and heart rate occur with K complexes, arousals, and large body movements.62, 63, 64 An age-related decrease in this response has been demonstrated in middle-aged subjects in comparison to younger subjects, possibly reflecting the decline in parasympathetic function that occurs with increasing age.65 Sharp increases in heart rate and blood pressure occur with morning awakening and beginning the day’s activities66,67 as well as after other periods of sleep, such as afternoon naps.66
REM sleep brings an increase in cardiovascular demands. Although blood pressure and heart rate have average levels near those observed in light NREM sleep or quiet wakefulness, their variability increases markedly during phasic REM sleep, with wide erratic fluctuations.59,68 These changes are related to bursts of increased sympathetic activity and to reduced vagal input to the heart.59,69 Cardiac efferent vagal tone and baroreceptor regulation are generally suppressed during REM sleep.59,68
Respiratory Function in Sleep
Sleep alters breathing patterns, ventilation, and arterial blood gas values. Periodic breathing, a cyclic waxing and waning of tidal volume, sometimes with brief apnea, is common at sleep onset in association with fluctuations between wakefulness and light sleep. Breathing is remarkably regular; however, in stable stage 2 and SWS. The pattern becomes faster and more erratic during phasic REM sleep.70
Minute ventilation falls, mainly because of reduced tidal volume, with an average decrease of approximately 0.4 to 1.5 L/min compared with quiet wakefulness.70 This hypoventilation leads to small changes in arterial blood gases, including a mild hypercapnia (increases of 3 to 7 mm/Hg in carbon dioxide tension), decreases of 0.01 to 0.06 units in pH, and a mild hypoxemia (decreases of 35 to 9.4 mm Hg in PaO2 and 2% or less in SaO2).70,71 Data regarding REM sleep are somewhat contradictory but suggest that minute ventilation, tidal volume, and respiratory frequency are similar to those in NREM sleep, with similar or somewhat greater changes in blood gas values.70,71 Other factors that contribute to hypoventilation in NREM sleep include reduced central drive to breathe caused by loss of the wakefulness stimulus and increased upper airway resistance to airflow due to reduced pharyngeal muscle tone.70,71 Tone in the intercostal muscles and diaphragm; however, is maintained. In REM sleep, tone is lost in both the intercostal and upper airway muscles, reducing the rib cage contribution to breathing and increasing upper airway resistance; however, the diaphragm is relatively spared from REMrelated paralysis. Phasic REM sleep also includes dysrhythmic changes in the stimulation of brainstem respiratory neurons, resulting in an erratic breathing pattern.70,71
The arterial blood gas changes stimulate an adaptive increase in ventilation, although the response is less effective in sleep than in wakefulness. In men, the ventilatory responses to both hypercapnia and hypoxemia decrease by approximately half from wakefulness to NREM sleep, with a further reduction in REM sleep. Women respond similarly to hypercapnia but somewhat differently to hypoxemia. Women have a lower ventilatory response than men when awake and little change in NREM sleep, but a similar fall in REM sleep.72,73
Arousal from sleep is a second adaptive response to blood gas changes because the change stimulates ventilation and permits voluntary action to cope with the situation, such as moving a pillow that interferes with breathing. Hypercapnia is a relatively effective arousal stimulus, awakening most healthy subjects before carbon dioxide tension rises 15 mm/Hg; concurrent hypoxemia enhances the response. Hypoxemia alone; however, is a poor arousal stimulus; study subjects often fail to awaken despite an SaO2 as low as 70%.70
Adaptive responses that protect the airway are less effective during sleep. Respiratory secretions are cleared less readily because of diminished mucociliary clearance,74 and the tendency to aspirate increases.75 In addition, sleep suppresses the cough reflex to irritating substances in the airways in both REM and NREM sleep.71
Thermoregulation in Sleep
Body temperature is regulated at a lower set point in NREM sleep than in wakefulness. In combination with reduced motor activity, this results in a decrease in temperature at sleep onset.36,47 The normal temperature-regulating mechanisms are markedly inhibited during REM sleep; during this stage of sleep, body temperature is influenced more by the environment than the hypothalamus. Body temperature also has an independent circadian rhythm that typically peaks in the late afternoon and reaches a minimum in the early morning hours of sleep.47
Sleep length depends on the phase of the circadian temperature rhythm at bedtime because the rising phase triggers awakening from sleep. A sleep period that begins when body temperature is low—for example, going to bed at 3:00 AM—is relatively short because temperature soon rises. In contrast, a sleep period that begins when temperature is high is relatively long because temperature drops and a subsequent rise in temperature does not occur for some time.47 The body temperature rhythm shifts a little earlier (phase advance) with aging, which may partly explain why many older people have an earlier wake-up time than younger adults.47,48
Cerebral Blood Flow in Sleep
Brain blood flow decreases in sleep in comparison to waking.76 Brain imaging studies reveal that there is a small to moderate decrease in brain blood flow in NREM sleep in the brainstem and cortex.77,78 In contrast, there is an marked increase in brain blood flow in REM sleep in the brain stem and limbic decrease, but a decrease in the frontal cortex.79 Poor performance on tasks after
sleep deprivation is associated with reduced metabolic activity in the frontal lobes, thalamus, and midbrain, which may be associated with the state of reduced alertness.80,81
sleep deprivation is associated with reduced metabolic activity in the frontal lobes, thalamus, and midbrain, which may be associated with the state of reduced alertness.80,81
Renal Function in Sleep
Urine flow is reduced and more concentrated during sleep with a decreased excretion of sodium, chloride, potassium, and calcium. The mechanisms involved in these changes are complex and include changes in renal blood flow, glomerular filtration, hormone secretion (vasopressin, aldosterone, prolactin, parathormone), and sympathetic neural stimulation.82 Because night-time potassium excretion is reduced, potassium infusions given at night may lead to higher serum levels than daytime infusions.83
From infancy to old age, males have penile erections (nocturnal penile tumescence) during REM sleep. Total tumescence time is greatest just before and during puberty and then may gradually decline. Sleep-related erectile activity can be monitored to aid in differentiating physical and psychological components of impotence.84
Endocrine Function in Sleep
Endocrine hormone secretion is influenced by sleep. For example, growth hormone secretion is highly sleep-dependent and most secretion occurs during the first few hours after sleep onset during SWS. If sleep is advanced or delayed, growth hormone secretion shifts accordingly. In contrast, thyroid hormone and cortisol secretions have independent circadian rhythms. Thyroid hormone secretion increases in the late evening; cortisol concentration increases in the latter half of the night and peaks toward the end of the normal sleep period or soon after awakening.85
The hormone melatonin, secreted by the pineal gland, induces sleepiness and has a marked circadian rhythm that is closely linked to the light-dark cycle, temperature, and cortisol rhythms. A late evening surge in melatonin begins at darkness, approximately 2 hours before bedtime, and is considered a marker of the body’s circadian timing system. Secretion peaks at approximately 3:00 AM but is suppressed by daylight to levels that are barely detectable. Bright light exposure suppresses melatonin secretion and can be used to help reset a person’s circadian clock.86
▪ CLINICAL EVALUATION, DIAGNOSIS, AND APPROACHES
There are three primary ways in which sleep and sleepiness are measured: subjectively, behaviorally, and objectively. In health, these measures are often, but not always, congruent; however, they become much less well associated when impaired sleep or other health problems are present.87, 88, 89, 90, 91, 92
Subjective Measurement
Subjective measures of sleep can be particularly useful for screening, triage, and assessing the effects of treatment.15,93 Types of information typically obtained include an individual’s assessment of sleep latency (time from lights out to the onset of sleep), number of awakenings, depth and length of sleep, refreshing quality of sleep, satisfaction with sleep, and soundness of sleep. This information can be collected through the use of sleep questionnaires, that is, the Pittsburgh Sleep Quality Index,94, 95, 96 St. Mary’s Hospital Sleep Questionnaire,97 Sleep Disorders Questionnaire,98 sleep diaries, visual analogue scales, Leed’s Sleep Evaluation Questionnaire,99 and interviews.93,100,101
Daytime sleepiness can also be measured using subjective measures such as the Epworth Sleepiness Scale, an instrument that has been widely used in the clinical and research settings.102, 103, 104, 105 Test scores greater than 10 or 11 have been reported in patients with sleep disorders that cause excessive daytime sleepiness (EDS); the average score for control subjects is 6 (possible range of scores is 0 to 24; higher score = greater subjective sleepiness).102,106 Although the results of studies examining the relationship between Epworth Sleepiness Scale scores and sleep apnea severity, for example, have been equivocal,11,107,108 improvement in scores after treatment of apnea with continuous positive airway pressure (CPAP) has been described.109,110 Thus, the instrument may be sensitive to and particularly helpful in evaluating clinical responses to interventions designed to improve sleep (Display 8-1).
Behavioral Assessment
Assessment of behaviors related to sleep and sleepiness are an important part of a thorough assessment. In fact, observation is considered the “gold standard” for sleep monitoring in infants.15 Typically, individuals who are sleepy or sleep-deprived manifest characteristic behaviors including yawning, eye rubbing, head nodding, ptosis of the eyelids, irritability, and slowed movement. Other observable waking behaviors that may be noted include automatic behavior, unintentional sleep episodes, cataplexy (a stereotypical feature of narcolepsy in which there is a sudden decrement in muscle tone and loss of deep tendon reflexes leading to muscle weakness, paralysis, and/or postural collapse), and sleep drunkenness.10 Although lying quietly in a horizontal position is typical, movements and position changes can occur and are a normal part of sleep behavior. Abnormal sleep-related behaviors include bizarre postures, restless sleep, jerking of the extremities, seizure activity, and dream enactment. Video recordings of these behaviors during polysomnography (PSG) are often made to assist in the assessment and diagnosis of sleep problems. Observations of patients’ nocturnal behaviors by health care providers, bed partners, or parents often play an important role in the diagnosis and treatment of sleep disorders.
Objective Measurement
The structure and timing of sleep stages and cycles can be studied objectively using PSG, a procedure involving the simultaneous recording of the EEG, the EMG, and the EOG. At the usual recording speed of 1 cm/s, a standard 30-cm page represents a 30-second period, or epoch. Each epoch is assigned a single sleep-stage score based primarily on changes in EEG frequency (in cycles/s, or hertz [Hz]) and amplitude (in microvolts [μV]), with confirmation by the EOG and EMG patterns.12 In addition to sleep-staging signals, PSG often includes the measurement of other physiological parameters, such as respiratory movements of the chest and abdomen, airflow at the nose and mouth, SaO2, electrocardiogram and leg movements (anterior tibialis EMG).
Daytime sleepiness (daytime sleep propensity or tendency) can be quantified using the Multiple Sleep Latency Test (MSLT).105 Beginning 1.5 to 2 hours at the end of a nocturnal polysomnographic recording, four to five 20-minute nap opportunities are typically given in 2-hour intervals. The sleep latency of any given nap
opportunity is defined as the time from lights out to the first 30-second epoch scored as sleep. The average sleep latency across all naps is calculated and expressed as the mean sleep latency. Possible mean sleep latency scores on the MSLT range from 0 to 20 minutes, with a low score indicating greater sleepiness. An MSLT score less than 5 minutes indicates pathological sleepiness and is a level at which patients often experience marked impairment of social and/or occupational functioning and at which they are generally advised against driving or operating heavy equipment.15,111 Scores between 5 and 10 minutes are considered to be in the “diagnostic gray zone,” whereas scores greater than 10 are considered normal.
opportunity is defined as the time from lights out to the first 30-second epoch scored as sleep. The average sleep latency across all naps is calculated and expressed as the mean sleep latency. Possible mean sleep latency scores on the MSLT range from 0 to 20 minutes, with a low score indicating greater sleepiness. An MSLT score less than 5 minutes indicates pathological sleepiness and is a level at which patients often experience marked impairment of social and/or occupational functioning and at which they are generally advised against driving or operating heavy equipment.15,111 Scores between 5 and 10 minutes are considered to be in the “diagnostic gray zone,” whereas scores greater than 10 are considered normal.

How likely are you to doze off or fall asleep in the following situations, in contrast to feeling just tired?
This refers to your usual way of life in recent times. Even if you have not done some of these things recently, try to work out how they would have affected you. Use the following scale to choose the most appropriate number for each situation:
0 = would never doze
1 = slight chance of dozing
2 = moderate chance of dozing
3 = high chance of dozing
Situation
Sitting and reading
Watching TV
Sitting, inactive in a public place (theater or meeting)
As a passenger in a car for an hour without a break
Lying down to rest in the afternoon when circumstances permit
Sitting and talking to someone
Sitting quietly after a lunch without alcohol
In a care, while stopped for a few minutes in traffic
Actigraphy is an alternative method sometimes used to objectively measure sleep/wake patterns by monitoring periods of activity and rest.112,113 Using a battery-operated wristwatch-size microprocessor that senses movement with a piezoelectric beam, continuous motion data can be obtained for long periods. Computer algorithms allow for analysis of activity and non-activity, as well as scoring of sleep and wakefulness.114 While actigraphy cannot determine sleep stages, information on total sleep time, percent of time spent awake, number of awakenings, time between awakenings, and sleep onset latency can be obtained. Actigraphy data correlate well with PSG data, particularly when sleep is normal.112,115 Correlations decrease when sleep is disturbed or activity is limited.114,116,117
Environmental Assessment
The environment can play an important role in the quantity and quality of sleep obtained. A thermoneutral environment (neither too hot nor too cold) is essential for normal thermoregulatory changes needed to optimize sleep.41 Excessive noise has also been shown to cause poor subjective and objective sleep measures.118, 119, 120, 121 Too much light exposure alters melatonin production.86 Nursing interventions themselves can significantly disturb sleep. Comfortable beds/mattresses, pillows, and nightwear facilitate sleep.122
▪ IMPAIRED SLEEP, SLEEP DISORDERS, AND EXCESSIVE DAYTIME SLEEPINESS
Impaired sleep can be generally categorized as either sleep deprivation (resulting from inadequate sleep) or sleep disruption (resulting from fragmented sleep during the night)123 (Fig. 8-6). Sleep deprivation frequently occurs in association with particular lifestyles or stages of development. Sleep disruption is often seen in health-related conditions. Both sleep deprivation and sleep disruption result in sleep loss.123 Important information has been obtained through sleep deprivation studies that have shown that sleep loss has numerous adverse effects including fatigue, anxiety, increase illness, increased sensitivity to pain, decreased immune response, restlessness, disorientation, decreased alertness/attention during the day, and decreased sense of well being.124, 125, 126, 127, 128, 129, 130 The results of several studies suggest that the duration of self-reported sleep time increases mortality, with those sleeping longer than 9 hours and less than 5 hours per night, had an increased risk of
mortality.131, 132, 133, 134, 135 However, the extent to which the duration of self-reported sleep is confounded by a number of demographic, behavioral, and psychological factors is unknown and further research is this area is greatly needed.136
mortality.131, 132, 133, 134, 135 However, the extent to which the duration of self-reported sleep is confounded by a number of demographic, behavioral, and psychological factors is unknown and further research is this area is greatly needed.136
![]() ▪ Figure 8-6 Causes and consequences of impaired sleep and targets of intervention in cardiovascular patients. |
Sleep disorders, specific diagnostic entities, include a wide array of problems characterized by insomnia (difficulty initiating or maintaining sleep or early morning awakening), EDS, and/or abnormal movements, behaviors, or sensations during sleep (Display 8-2).10 There are eight groups of primary sleep disorders outlined in the International Classification of Sleep Disorders,10 including the insomnias, sleep-related breathing disorders, hypersomnias of central origin, circadian rhythm disorders, parasomnias, sleep-related movement disorders, normal sleep variants, and “other” (physiological, nonphysiological, and environmental sleep disorders).
EDS, the inability to maintain the alert awake state, is the most common consequence of sleep disorders and/or insufficient or poor sleep and is the most prevalent symptom of patients seen in sleep disorders centers in the United States.13 However, because of its often vague and nonspecific clinical presentation, the condition is frequently unrecognized by health care providers. Patients themselves may have very little insight into the nature and severity of the problem and the negative effects that EDS has on their lives. For in its milder forms, EDS may cause only minor, barely perceived decrements in social and occupational functioning. When severe; however, it can be debilitating, causing a broad range of neuropsychological deficits affecting daytime functioning and quality of life. EDS can even be life threatening because of associated alterations in alertness and reactivity.124, 125, 126, 127, 128, 129, 130 Increased napping has also been associated with increased mortality in the elderly.137,138

Insomnia
Adjustment insomnia (acute Insomnia)
Psychophysiological insomnia
Paradoxical insomnia
Idiopathic insomnia
Insomnia due to mental disorder
Inadequate sleep hygiene
Behavioral insomnia of childhood
Insomnia due to drug or substance
Insomnia due to medical condition
Insomnia not due to substance or known physiological condition, unspecified (nonorganic insomnia, NOS)
Physiological (organic) insomnia, unspecified
Sleep-Related Breathing Disorders
Central Sleep Apnea Syndromes
Primary central sleep apnea
Central sleep apnea due to Cheyne-Stokes breathing pattern
Central sleep apnea high-altitude periodic breathing
Central sleep apnea due to medical condition not Cheyne-Stokes
Central sleep apnea due to drug or substance
Primary sleep apnea of infancy (formerly primary sleep apnea of newborn)
Obstructive Sleep Apnea Syndromes
Obstructive sleep apnea, adult
Obstructive sleep apnea, pediatric
Sleep-Related Hypoventilation/Hypoxemic Syndromes
Sleep-related nonobstructive Aaveolar hypoventilation, idiopathic
Congenital central alveolar hypoventilation syndrome
Sleep-Related Hypoventilation/Hypoxemia Due to Medical Condition
Sleep-related hypoventilation/hypoxemia due to pulmonary parenchymal or vascular pathology
Sleep-related hypoventilation/hypoxemia due to lower airways obstruction
Sleep-related hypoventilation/hypoxemia due to neuromuscular and chest wall disorders
Other Sleep-Related Breathing Disorder
Sleep apnea/sleep-related breathing disorder, unspecified
Hypersomnias of Central Origin Not Due to a Circidian Rhythm Sleep Disorder, Sleep-Related Breathing Disorder, or Other Cause of Disturbed Nocturnal Sleep
Narcolepsy with cataplexy
Narcolepsy without cataplexy
Narcolepsy due to medical condition
Narcolepsy, unspecified
Recurrent hypersomnia Kleine-Levin syndrome Menstrual-related hypersomnia
Idiopathic hypersomnia with long sleep time
Idiopathic hypersomnia without long sleep time
Behaviorally induced insufficient sleep syndrome
Hypersomnia due to medical condition
Hypersomnia due to drug or substance
Hypersomnia not due to substance or known physiological condition (nonorganic hypersomnia, NOS)
Physiological (organic) hypersomnia, unspecified (organic hypersomnia, NOS)
Circadian Rhythm Sleep Disorders
Circadian rhythm sleep disorder, delayed sleep phase type (delayed sleep phase disorder)
Circadian rhythm sleep disorder, advanced sleep phase type (advanced sleep phase disorder)
Circadian rhythm sleep disorder, irregular sleep-wake type (irregular sleep-wake rhythm)
Circadian rhythm sleep disorder, free-running type (nonentrained type)
Circadian rhythm sleep disorder, jet lag type (jet lag disorder)
Circadian rhythm sleep disorder, shift work type (shift work disorder)
Circadian rhythm sleep disorder due to medical condition
Other circadian rhythm sleep disorder (circadian rhythm disorder, NOS)
Other circadian rhythm sleep disorder due to drug or substance
Parasomnias
Disorders of Arousal (From NREM Sleep)
Confusional arousals
Sleepwalking
Sleep terrors
Parasomnias Usually Associated With REM Sleep
REM sleep behavior disorder (including parasomnia overlap disorder and status dissociatus)
Recurrent isolated sleep paralysis
Nightmare disorder
Other Parasomnias
Sleep-related dissociative disorders
Sleep enuresis
Sleep related groaning (catathrenia)
Exploding head syndrome
Sleep-related hallucinations
Sleep-related eating disorder
Parasomnia, unspecified
Parasomnia due to drug or substance
Parasomnia due to medical condition
Sleep-Related Movement Disorders
Restless legs syndrome
Periodic limb movement disorder
Sleep-related leg cramps
Sleep-related bruxism
Sleep-related rhythmic movement disorder
Sleep-related movement disorder, unspecified
Sleep-related movement disorder, due to drug or substance
Sleep-related movement disorder due to medical condition
Isolated Symptoms, Apparently Normal Variants and Unresolved Issues
Long sleeper
Short sleeper
Snoring
Sleep talking
Sleep starts (hypnic Jerks)
Benign sleep myoclonus of infancy
Hypnagogic foot tremor and alternating leg muscle activation during sleep
Propriospinal myoclonus at sleep onset
Excessive fragmentary myoclonus
Other Sleep Disorders
Other physiological (organic) sleep disorder
Other sleep disorder not due to substance or known physiological condition
Environmental sleep disorder
Propriospinal myoclonus at sleep onset
Excessive fragmentary myoclonus
Other Sleep Disorders
Other physiological (organic) sleep disorder
Other sleep disorder not due to substance or known physiological condition
Environmental sleep disorder
Appendix A: Sleep Disorders Associated With Conditions Classifiable Elsewhere
Fatal familial insomnia
Fibromyalgia
Sleep-related epilepsy
Sleep-related headaches
Sleep-related gastroesophageal reflux disease
Sleep-related coronary artery ischemia
Sleep-related abnormal swallowing, choking, and laryngospasm
Appendix B: Other Psychiatric and Behavioral Disorders Frequently Encountered in the Differential Diagnosis of Sleep Disorders
Mood disorders
Anxiety disorders
Somatoform disorders
Schizophrenia and other psychotic disorders
Disorders usually first diagnosed in infancy, childhood, or adolescence
Personality disorders
From AASM. (2005). The international classification of sleep disorders. Westchester, IL: American Academy of Sleep Medicine.
SLEEP-RELATED DISORDERED BREATHING
Sleep-related changes in breathing and oxygenation have important cardiovascular consequences. This section focuses on the cardiovascular impact of sleep in obstructive sleep apnea (OSA), central sleep apnea (CSA), and snoring/upper airway resistance syndrome (UARS).
Obstructive Sleep Apnea
Patients with sleep apnea repeatedly stop breathing during sleep for periods of 10 seconds or longer (Fig. 8-7).10 Apnea can be obstructive (a collapsed upper airway blocks airflow despite effort to breathe), central (no respiratory effort), or mixed (central, then obstructive component). A predominance of obstructive apnea is the most common pattern and can lead to repetitive episodes of hypoxemia that are terminated by brief arousals. Typical patients
are middle-aged men who are overweight, snore loudly during sleep, and experience daytime sleepiness that interferes with normal activities; women with sleep apnea are usually postmenopausal. Other risk factors include a positive family history and race (African Americans, Mexican Americans, Pacific Islanders, and East Asians).139, 140, 141 Sleep apnea is likely to be worsened by sleep deprivation,142, 143, 144 alcohol ingestion,141,145, 146, 147 and sedative or hypnotic use.148
are middle-aged men who are overweight, snore loudly during sleep, and experience daytime sleepiness that interferes with normal activities; women with sleep apnea are usually postmenopausal. Other risk factors include a positive family history and race (African Americans, Mexican Americans, Pacific Islanders, and East Asians).139, 140, 141 Sleep apnea is likely to be worsened by sleep deprivation,142, 143, 144 alcohol ingestion,141,145, 146, 147 and sedative or hypnotic use.148
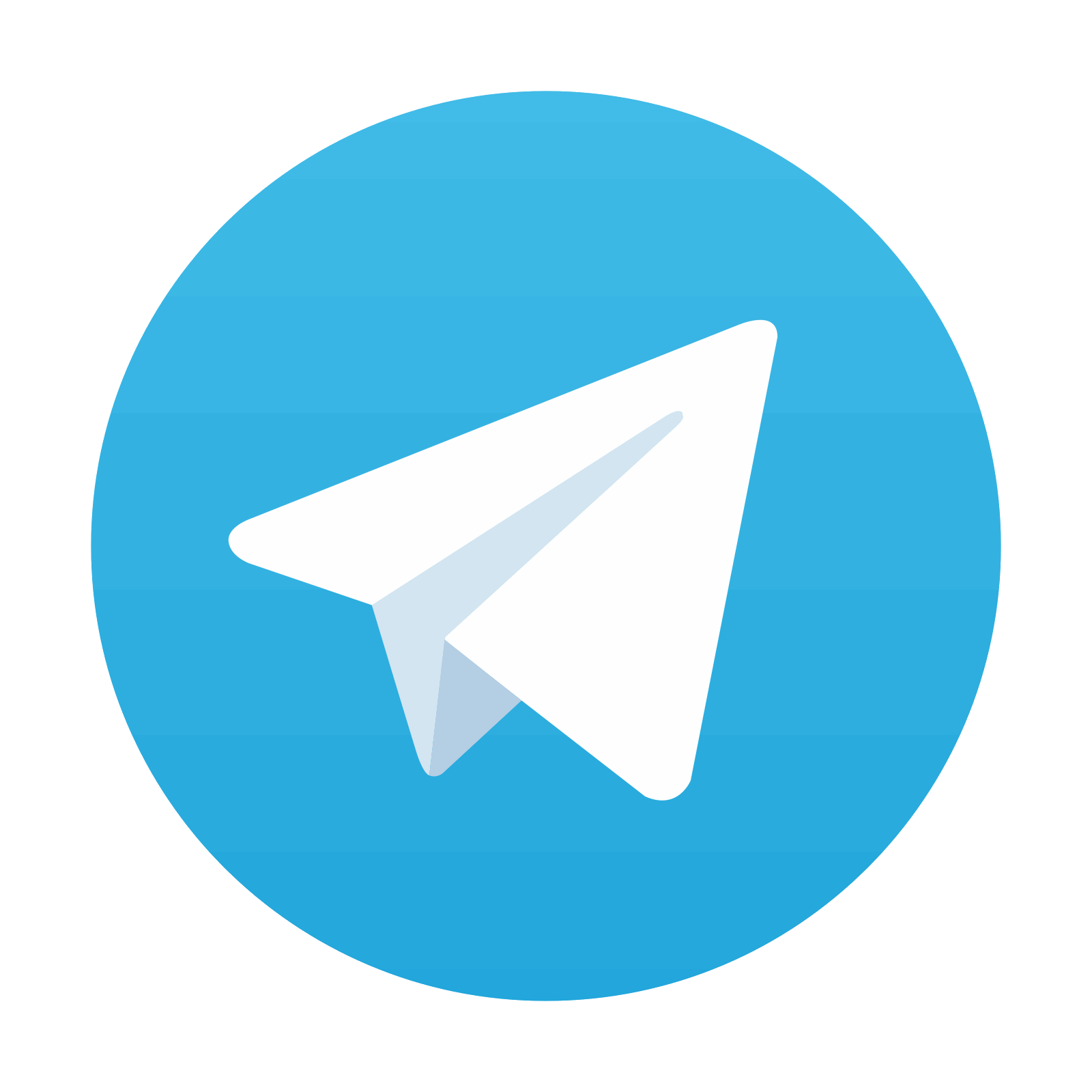
Stay updated, free articles. Join our Telegram channel

Full access? Get Clinical Tree
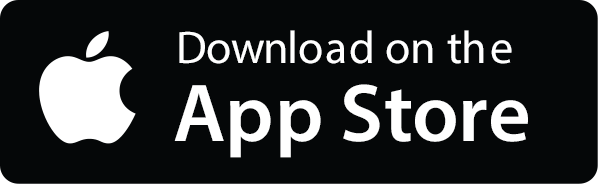
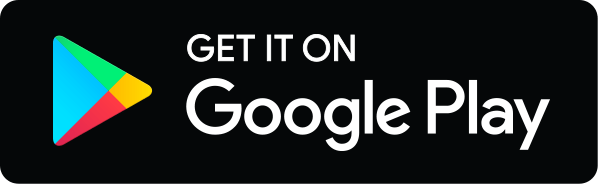
Get Clinical Tree app for offline access
