|
other immune system molecules are responsible for regulating acute-phase proteins; they also produce changes in other cells that cause systemic symptoms of inflammation, such as anorexia, fever, lethargy, and weight loss. Clinical and laboratory findings used to identify the presence of inflammation are listed in Box 16.1.
Decreased serum albumin
Decreased serum transferrin
Decreased serum prealbumin
Increased C-reactive protein
Increased blood glucose
Increased or decreased WBC count
Increased percentage of neutrophils in the cell differential
Decreased platelet count
Negative nitrogen balance
Fever
Hypothermia
Presence of infection
Urinary tract infection
Pneumonia
Blood stream infection
Wound or incision infection
Abscess
Weight loss, such as 1% to 2% of usual body weight in 1 week
Calorie intake of <75% for >7 days
Mild depletion of body fat
Mild depletion of muscle mass
Mild fluid accumulation
EN is strongly recommended over PN when appropriate.
EN should start within 24 to 48 hours following admission.
Either gastric or small bowel feedings are appropriate; consider small bowel feedings if patient is at high risk for aspiration or has intolerance to gastric feedings.
Calorie needs can be determined by indirect calorimetry, predictive equations, or by a simple weight-based formula:
25 to 30 cal/kg/day of admission weight for patients with BMI <30
11 to 14 cal/kg of actual body weight per day for patients with BMI in the range of 30-50
22 to 25 cal/kg of ideal body weight per day for patients with BMI >50
Positive outcomes in critical illness may be more dependent on adequate protein than adequate total calorie intake.
Protein recommendations are
1.2 to 2.0 g/kg/actual weight for adults with BMI <30; higher amounts may be necessary for certain illnesses, such as burns
≥2.0 g/kg ideal body weight per day for patients with BMI of 30 to 40
Up to 2.5 g/kg ideal body weight per day for patients with BMI ≥40
Supplemental glutamine added to an EN formula not already containing glutamine is not recommended.
Antioxidant micronutrients, such as vitamins E and C, selenium, zinc, and copper, may improve outcomes for patients with burns, trauma, and those requiring mechanical ventilation.
Fluid requirements are highly individualized according to losses that occur through exudates, hemorrhage, emesis, diuresis, diarrhea, and fever.
Patients with persistent diarrhea may benefit from the use of the fiber-containing formula, a semi-elemental formula in place of a polymeric formula, or a soluble fiber supplement.
at high risk for aspiration or those who are intolerant to gastric feedings. Volume-based feeding protocols that specify total amount per 24 hours provide more nutrition than hourly rates (McClave et al., 2016).
Initiate PN after 7 days of hospitalization when EN is not feasible in patients at low nutrition risk.
Initiate PN as soon as possible after admission to the ICU when EN is not feasible in patients at high nutrition risk or those who are malnourished.
Consider supplemental PN after 7 to 10 days in patients who are unable to meet >60% of their calorie and protein requirements via EN regardless of the patients’ level of nutrition risk.
Initiate EN as soon as possible in patients on PN. PN is discontinued when EN provides >60% of the patient’s estimated calorie requirements.
For most patients, a standard polymeric (intact macronutrients) formula may be used to initiate nutrition support in the ICU.
For obese patients, a low-caloric density formula with a reduced nonprotein calorie-to-nitrogen ratio (NPC:N) is suggested (proportionately lower in calories from carbohydrates and fats than in protein content).
For patients in the surgical ICU or with severe trauma, an immune-modulating formula that provides arginine, fish oils, and/or glutamine may be considered.
No benefit has been shown for routine use of other specialty formulas and disease-specific formulas in the ICU, such as pulmonary formulas for acute respiratory failure and hepatic formulas for critically ill patients with acute or chronic liver disease.
by Peake et al. (2014) found that substituting routine formula that provides 1.0 cal/mL with a 1.5-cal/mL formula resulted in 46% greater delivery of calories without adverse effects when administered at the same rate. Studies are needed to determine if increasing the delivery of calories affects patient outcomes.
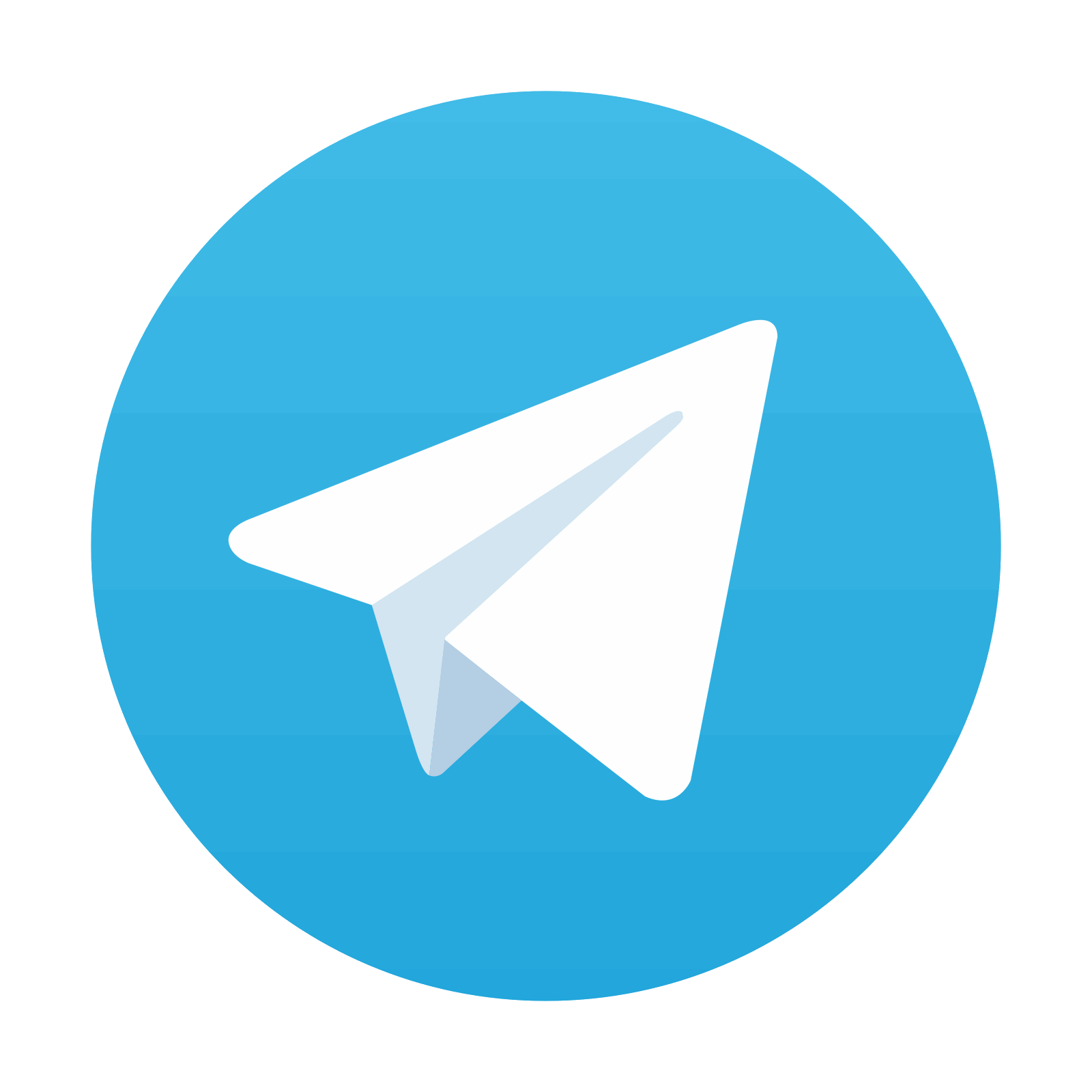
Stay updated, free articles. Join our Telegram channel

Full access? Get Clinical Tree
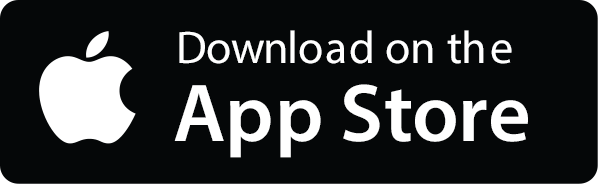
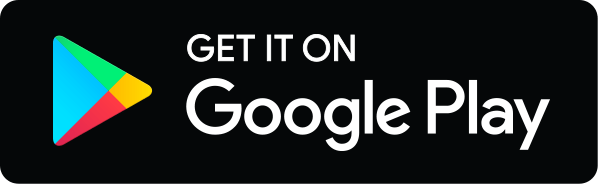