Nuclear, Magnetic Resonance, and Computed Tomography Imaging
Laurie A. Soine
Peter J. Cawley
Many advances have been made in noninvasive cardiovascular imaging over the last 20 years. Fueled by scientific advances in the fields of computer technology, processing and storing images, physics, biochemistry, and engineering noninvasive cardiovascular imaging has become a widely available clinical tool. Nuclear cardiology, magnetic resonance imaging (MRI), computed tomography (CT), and ultrasound (echocardiography, or echo) are different imaging modalities able to create tomographic images of cardiovascular structures. A tomogram is an imaging “section” or “slice” of an object created by an imaging modality.

Radionuclides, substances that emit radioactivity, have been used as a tracer in the body for more than 65 years. Since the development of the γ-ray camera by Anger some 30 years ago and the introduction of radioactive potassium analogs, the use of radionuclides (radiotracers) to study the heart has been the subject of increasing clinical application. The introduction of new and improved radioisotopes and imaging techniques in the 1980s led to widespread clinical application.
Radioisotope Pharmaceuticals
Radionuclides are atoms in an unstable form. They have a finite probability of spontaneously converting to a more stable configuration. When they do so, small amounts of energy in the form of rays are emitted. The rate at which atoms in a given sample undergo this conversion is denoted by the half-life, the time required for one half of the sample to undergo the conversion. Half-lives of radioactive substances vary from a fraction of a second to a millennia; the half-life for any given radionuclide is always the same. The characteristics of an ideal radiotracer to assess myocardial blood flow would include: a half-life long enough to allow for convenient imaging, easy combination with biologic substances, 100% myocardial extraction across the entire spectrum of achievable or inducible coronary blood flow states, instantaneous intracellular binding, low extraction and clearance by organs adjacent to the heart, and extraction by only viable cells. Unfortunately, to date, no commercially available radiotracer of myocardial perfusion meets all of these criteria.
Single-photon emission computed tomography (SPECT) and positron emission tomography (PET) are the two most common nuclear cardiovascular imaging modalities. The three most common radiotracers used in SPECT imaging are thallium 201 (201Tl) and the two technetium 99m-labeled tracers: 99mTcsestamibi (Cardiolite) and 99mTc-tetrofosmin (Myoview). Nitrogen-13-ammonia, oxygen-15 labeled water, and rubidium-82 are the most common tracers used in PET imaging to assess blood flow; fluorine-18-2-fluoro-2-deoxyglucose is used in PET imaging to assess myocardial metabolism.
Injection and detection of these radiotracers allow for the measurement of relative myocardial blood flow. The radiotracers follow blood flow and are extracted from the blood pool by myocytes in proportion to blood flow. The mechanism by which the various radiotracers, used in SPECT and PET imaging, are extracted from the blood stream and taken up by myocytes varies. In SPECT imaging, the radioactive decay of tracer is detected outside the body as scintillation (flash of light) by a gamma scintillation camera. The camera functions as a scanning device (large Geiger counter) to detect the distribution of radioactivity emitting from the myocardium through the chest wall, allowing examination of myocardial structure and function.201Tl is a potassium analog. Because of the dynamic equilibrium of potassium between cells and the blood pool, potassium and therefore201Tl, distributes in the myocardium in proportion to the blood flow. Myocytes with an intact sodium-potassium pump take up201Tl. However, the relatively low energy (60 keV) emitted by201Tl makes imaging of this tracer suboptimal in large patients. The technetium 99m-labeled radiotracers passively distribute across sarcolemmal and mitochondrial membranes and remain intracellularly bound. The relatively higher energy (140 keV) emitted by this group of tracers allows for improved transmission through the chest tissue resulting in improved image quality, particularly in large patients.
PET imaging likewise allows for quantitative and tomographic imaging of myocardial perfusion and metabolism without intrinsically altering these processes. A positron-emitting radiotracer travels only a short distance in tissue prior to encountering an electron. This interaction causes annihilation of both particles, producing two high-energy photons that depart at an angle of 180 degrees from each other. PET imaging systems are designed to detect the two photons, which travel in opposite directions at essentially the speed of light (511 keV). By measuring the time that it takes for each photon to encounter the circumferential ring of detectors, localization of the event can be mathematically derived.
Diagnostic Indications for Nuclear Imaging
Table 14-1 summarizes the diagnostic uses of the imaging modalities discussed in this chapter. Stress myocardial perfusion with SPECT and PET imaging is useful clinically for the detection of flow-limiting coronary artery disease and risk stratification for patients with known coronary heart disease.1 The clinical aim of these studies is to evaluate the physiology of coronary blood flow. Myocardial perfusion images provide the clinician and patient
with important predictive and prognostic information, including left ventricular (LV) chamber size, and global and regional LV function; location, size, and extent of impairment of coronary flow reserve (myocardial ischemia); and location, size, and extent of myocardial infarction.2
with important predictive and prognostic information, including left ventricular (LV) chamber size, and global and regional LV function; location, size, and extent of impairment of coronary flow reserve (myocardial ischemia); and location, size, and extent of myocardial infarction.2
Table 14-1 ▪ CLINICAL APPLICATION OF TOMOGRAPHIC IMAGING MODALITIES | |||||||||||||||||||||||||||||||||||||||||||||||||||||||||||||||||||||||||
---|---|---|---|---|---|---|---|---|---|---|---|---|---|---|---|---|---|---|---|---|---|---|---|---|---|---|---|---|---|---|---|---|---|---|---|---|---|---|---|---|---|---|---|---|---|---|---|---|---|---|---|---|---|---|---|---|---|---|---|---|---|---|---|---|---|---|---|---|---|---|---|---|---|
|
Stress myocardial perfusion imaging involves comparing the pattern of myocardial blood flow, as reflected by myocyte uptake of radiotracer, in a resting state and in the hyperemic or stress state. The goals of stress perfusion imaging are creating heterogeneity of myocardial blood flow, marking this effect with a radiotracer, and applying SPECT or PET to obtain images of myocardial uptake.
The most common stress myocardial perfusion study using SPECT imaging is the “dual” (two) isotope imaging protocol. This protocol uses201Tl to evaluate the pattern of myocardial blood flow at rest and a technetium 99m-labeled product (sestamibi or tetrofosmin) to obtain the stress images. However, increasingly low doses of technetium 99m-labeled products are injected at rest followed by higher doses at peak stress. Regardless of the radiotracer used, the premise remains the same—obtaining images of myocardial blood flow at rest and comparing these images to a pattern of radiotracer myocardial uptake in the stressed state. Thus, all patients receive an intravenous (IV) injection of radiotracer while sitting quietly at rest. A gated set of SPECT or PET myocardial perfusion images are then immediately acquired. After acquisition of the images at rest, the patient is “stressed,” or rather a hyperemic state of coronary blood flow is created, and this state is marked with a second radiotracer injection. A patient reaches the stress state by exercising on the treadmill, and achieving 85% of the maximal predicted heart rate for age (220 − patient’s age × 0.85). In patients with normal coronary arteries, as the heart rate and blood pressure rise in response to exercise, normal coronary arteries dilate. Coronary flow reserve is maintained in arteries with less than 70% stenosis.3 In contrast, a region of the myocardium supplied by a diseased artery is unable to appropriately dilate in response to increasing myocardial work resulting in a relative reduction in blood flow/radiotracer uptake compared with normal coronary arteries. Studies of myocardial blood flow have demonstrated that at a heart rate of 85% of maximal predicted for age, blood flow through a normal coronary artery increases approximately two-fold. Therefore, at peak exercise, regions of the myocardium supplied by normal coronary arteries receive two-fold the amount of radiotracer compared with regions of the myocardium supplied by diseased vessels, unable to dilate. In patients unable to exercise adequately, an IV infusion of dipyridamole (0.57 mg/kg infused over 4 minutes) or adenosine (140 mcg/kg/min) reproduce this hyperemic state. The vasodilator agents do not stress the myocardium but rather dilate normal coronary arteries, having little effect on diseased vessels. At the standard infusion doses and rates, these agents dilate normal coronary arteries four- to five-fold.4 Following the stress study, a second set of gated SPECT or PET images are acquired.
The rest and post-stress gated SPECT images when processed and reconstructed, reflect the pattern of myocardial blood flow in the two states. The images are reconstructed in standardized views (short, vertical, and horizontal long axis). Patterns of radiotracer uptake are compared between the two datasets. A segmental scoring system has become a widely applied quantitative tool to integrate the extent and severity of perfusion abnormalities.5 Summed stress score is a quantitative tool used to evaluate the extent and severity of myocardium at risk.6 The score is determined by dividing the myocardium into 17 segments, scoring the percent reduction in radiotracer uptake, and adding the segments. The 17-segment representation of the myocardium is a standard format used in most myocardial imaging modalities.
A segment or region of the myocardium that has reduced radiotracer uptake on the images obtained at stress state that appears more uniform (improved) on the images obtained at rest is consistent with myocardial ischemia. The severity of the impairment of coronary flow reserve can be qualitatively estimated by the degree of reduction of radiotracer uptake (i.e., mildly reduced, moderately reduced, or severely reduced uptake). A segment or region of the myocardium with a fixed (i.e., present on both rest and stress) reduction in radiotracer uptake on both stress and rest images is most consistent with myocardial injury or infarction. An alternative explanation of an apparent fixed region of reduced radiotracer uptake that thickens and moves normally on gated cines may be attenuation artifact. The most common attenuating structures include breast tissue (anterior wall) and diaphragm (inferior wall). A reversible abnormality (present on stress, not on rest) is consistent with myocardial ischemia (Fig. 14-1).
The summed stress score (extent and severity of ischemia and infarction) when combined with Duke Treadmill Score has been shown to be an independent predictor of cardiac death7 (Fig. 14-2). In addition to increasing the identification and quantification of coronary artery disease, stress myocardial perfusion data have been shown to be an independent additional predictor of serious cardiac events in the ensuing year in men and women of all ages, as well as patients with diabetes.2,7, 8, 9, 10, 11
Stress myocardial perfusion using PET imaging provides a more quantitative measurement of coronary blood flow. By comparing absolute blood flow at rest with absolute flow in a hyperemic state created by a vasodilator infusion, impairment of coronary flow reserve can be more precisely measured. PET imaging allows for precise detection of global impairment in coronary flow reserve and therefore may increasingly be useful
in the diagnosis of microvascular disease. Several of the radiotracers used in PET imaging require an on-site cyclotron for radiotracer generation. Thus, widespread application of PET imaging is currently limited by the cost associated with generating the radiotracers.
in the diagnosis of microvascular disease. Several of the radiotracers used in PET imaging require an on-site cyclotron for radiotracer generation. Thus, widespread application of PET imaging is currently limited by the cost associated with generating the radiotracers.
Myocardial Viability
LV dysfunction and associated heart failure is increasing in both incidence and prevalence in the United States. The mortality and morbidity associated with LV dysfunction and heart failure are high.12 While dramatic advances in medical therapy have resulted in improved survival and functional capacity, the best and most definitive therapy, when appropriate, is revascularization. Coronary artery disease accounts for approximately two thirds of cases of heart failure in the United States.13 Imaging studies to assess myocardial viability help identify patients who may benefit from revascularization. Myocardial viability is described as a condition of chronic sustained abnormal contraction of the myocardium secondary to chronic underperfusion, in patients with known coronary artery disease and in whom revascularization results in recovery of LV function.14 LV dysfunction may not be the result of irreversible scar but rather caused by impairment in function and energy use of viable myocytes—myocytes that if fueled with adequate blood flow would demonstrate improved function. Chronic myocardial ischemia is associated with a severe reduction in contractile function. Patients with LV dysfunction who have viable myocardium are at highest risk of cardiac death, but at the same time benefit most from revascularization.15, 16, 17, 18 In contrast to infarct-related scar, dysfunctional but viable myocardium has the potential to regain function.19
201Tl combined with SPECT imaging has historically been the most common radionuclide used for distinguishing viable or hibernating myocardium from scar.201Tl is injected in the resting state; gated SPECT images immediately acquired reflect myocardial perfusion. Delayed images acquired 4 to 24 hours later represent tissue metabolism. A region of the myocardium with reduced perfusion (resting thallium uptake) but preserved metabolism (improved thallium uptake on delay images), defines myocardial viability.20 Myocardial viability studies with201Tl are clinically helpful in patients with multivessel coronary artery disease and LV dysfunction. Potential reversibility of LV dysfunction is an important clinical consideration in these patients. Multiple studies have demonstrated that patients with viable myocardium benefit from revascularization versus augmented medical therapy. Alternatively, patients without evidence of viability do not show this same improvement after revascularization.21,22
PET imaging is emerging as a precise method for the detection of myocardial viability. The use of fluorine-18-2-fluoro-2-deoxyglucose (FDG) to assess myocardial metabolism and nitrogen-13-ammonia to assess perfusion, result in a highly sensitive study for differentiating viable myocardium from scar.23,24 A perfusion-metabolism mismatch pattern has become synonymous with reversible contractile dysfunction and thus prediction of improvement in LV function after revascularization.25
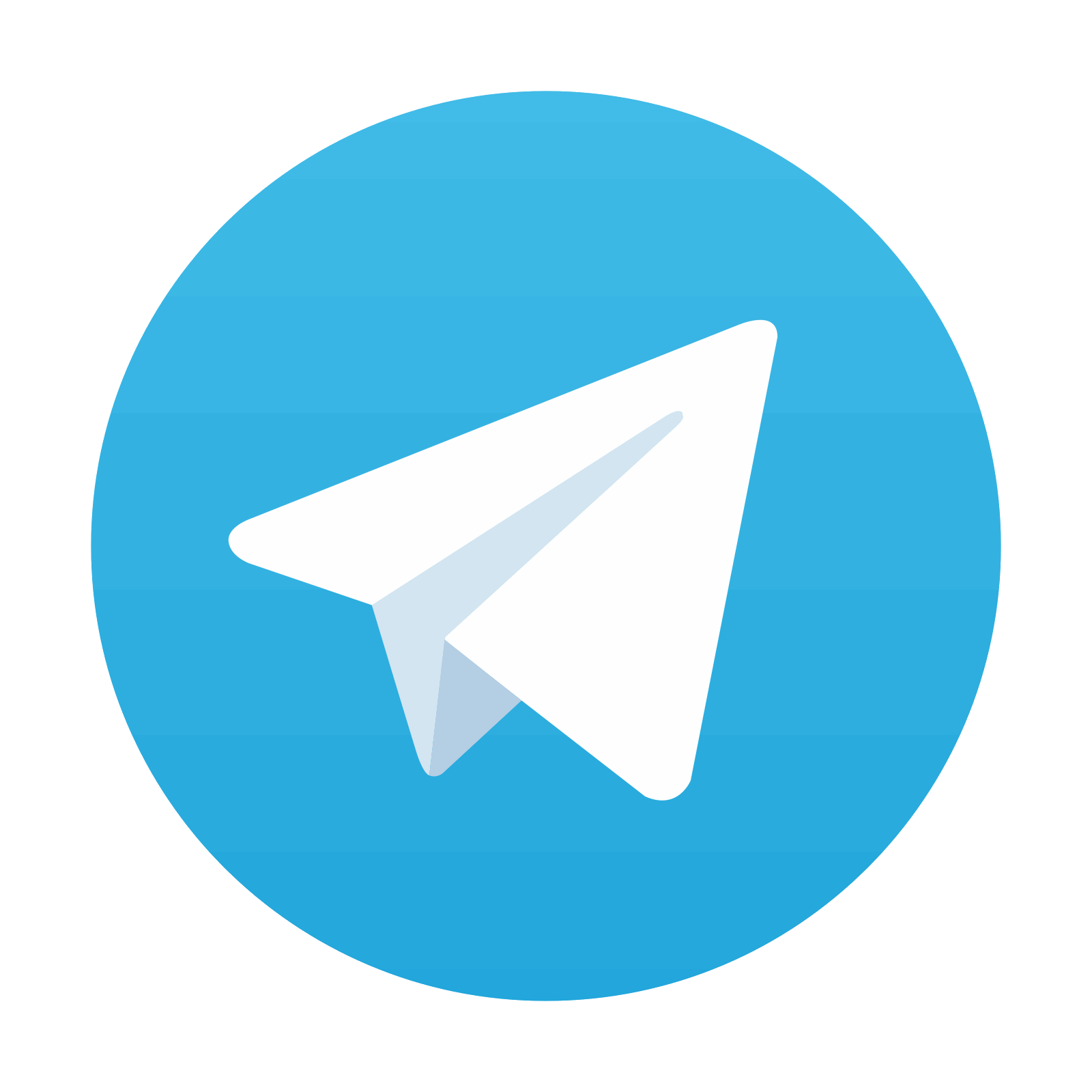
Stay updated, free articles. Join our Telegram channel

Full access? Get Clinical Tree
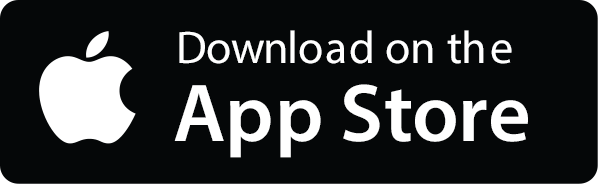
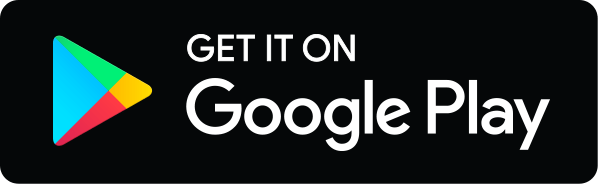