
been identified: apo A-I, apo A-II, apo B-100, apo B-48, apo C-I, apo C-II, apo C-III, apo E2, apo E3, apo E4, and lipoprotein(a), or Lp(a). In addition, the actions of several lipoprotein-processing enzymes (lipoprotein lipase [LPL], hepatic lipase [HL], lecithin cholesterol acyltransferase, and cholesteryl ester transfer protein [CETP]) and the function of cell receptors, including the LDL and chylomicron remnant receptor, are now established. These advances permit an understanding of lipid metabolism, as well as the abnormalities leading to elevated blood cholesterol.
Table 36-1 ▪ SELECTED, RANDOMIZED, CLINICAL TRIALS USING STATIN THERAPY TO LOWER CHOLESTEROL | |||||||||||||||||||||||||||||||||||||||||||||||||||||||||||||||||||||||||||||||||||||||||||||||||||||||||||||||||||||||||||||||||||||||||||||||||||||||||||||||||||||||||||
---|---|---|---|---|---|---|---|---|---|---|---|---|---|---|---|---|---|---|---|---|---|---|---|---|---|---|---|---|---|---|---|---|---|---|---|---|---|---|---|---|---|---|---|---|---|---|---|---|---|---|---|---|---|---|---|---|---|---|---|---|---|---|---|---|---|---|---|---|---|---|---|---|---|---|---|---|---|---|---|---|---|---|---|---|---|---|---|---|---|---|---|---|---|---|---|---|---|---|---|---|---|---|---|---|---|---|---|---|---|---|---|---|---|---|---|---|---|---|---|---|---|---|---|---|---|---|---|---|---|---|---|---|---|---|---|---|---|---|---|---|---|---|---|---|---|---|---|---|---|---|---|---|---|---|---|---|---|---|---|---|---|---|---|---|---|---|---|---|---|---|---|
|
Table 36-2 ▪ GUIDELINES FOR INITIATION OF TLC AND/OR PHARMACOTHERAPIES—MODIFICATIONS BASED ON THE UPDATE TO ATP III3 | |||||||||||||||||||||||||||||||||||||||||||||||
---|---|---|---|---|---|---|---|---|---|---|---|---|---|---|---|---|---|---|---|---|---|---|---|---|---|---|---|---|---|---|---|---|---|---|---|---|---|---|---|---|---|---|---|---|---|---|---|
|

inhibited LDL receptor activity. High LDL levels also can result from a decrease in clearance of LDL because of a deficiency in LDL receptors. This deficiency may be caused by genetic abnormalities in the structure of the receptor binding sites (where apolipoproteins bind) or by a decrease in LDL receptors on the surface of cells. In addition, genetic mutation in apoproteins, particularly apo E and apo B-100, can result in decreased cholesterol clearance. The metabolic consequence is an increased blood level of this atherogenic lipoprotein and the synthesis of cholesterol within cells, a process normally suppressed by LDL uptake.
![]() ▪ Figure 36-1 The exogenous metabolism of lipoproteins and the transport of chylomicrons to the tissues and chylomicron remnants to the liver. |

Production of apo A-I is higher in women than in men and is increased by exercise training, alcohol consumption, and estrogen administration.21 Premenopausal women have more than three times the concentration of HDL2 than do men. Studies have also suggested that HDL may act as an antioxidant, preventing the oxidation of LDL.45,46




observed abnormality. A high intake of dietary cholesterol and saturated fatty acids downregulates LDL receptor activity and receptor synthesis, resulting in decreased LDL clearance.63
Table 36-3 ▪ LIPID ABNORMALITIES AND ASSOCIATED MECHANISMS | ||||||||||||||||||||||||||||||||||||||||||||||||||||
---|---|---|---|---|---|---|---|---|---|---|---|---|---|---|---|---|---|---|---|---|---|---|---|---|---|---|---|---|---|---|---|---|---|---|---|---|---|---|---|---|---|---|---|---|---|---|---|---|---|---|---|---|
|
VLDL. LDL cholesterol is more glycated in patients with diabetes compared with nondiabetic subjects. Glycated LDL particles have increased oxidative susceptibility.65 HDL is often low in patients with diabetes as a result of increased HL triglyceride activity. Additionally, hyperglycemia is associated with significantly increased mortality in patients with acute coronary syndrome. The Heart Protection Study (HPS) further confirmed the importance of lipid management in persons with type 2 diabetes. HPS included 5,963 persons with diabetes (ages 40 to 80 years). Those subjects receiving simvastatin 40 mg/day had significant reductions of 25% for major coronary events including stroke and revascularization.12
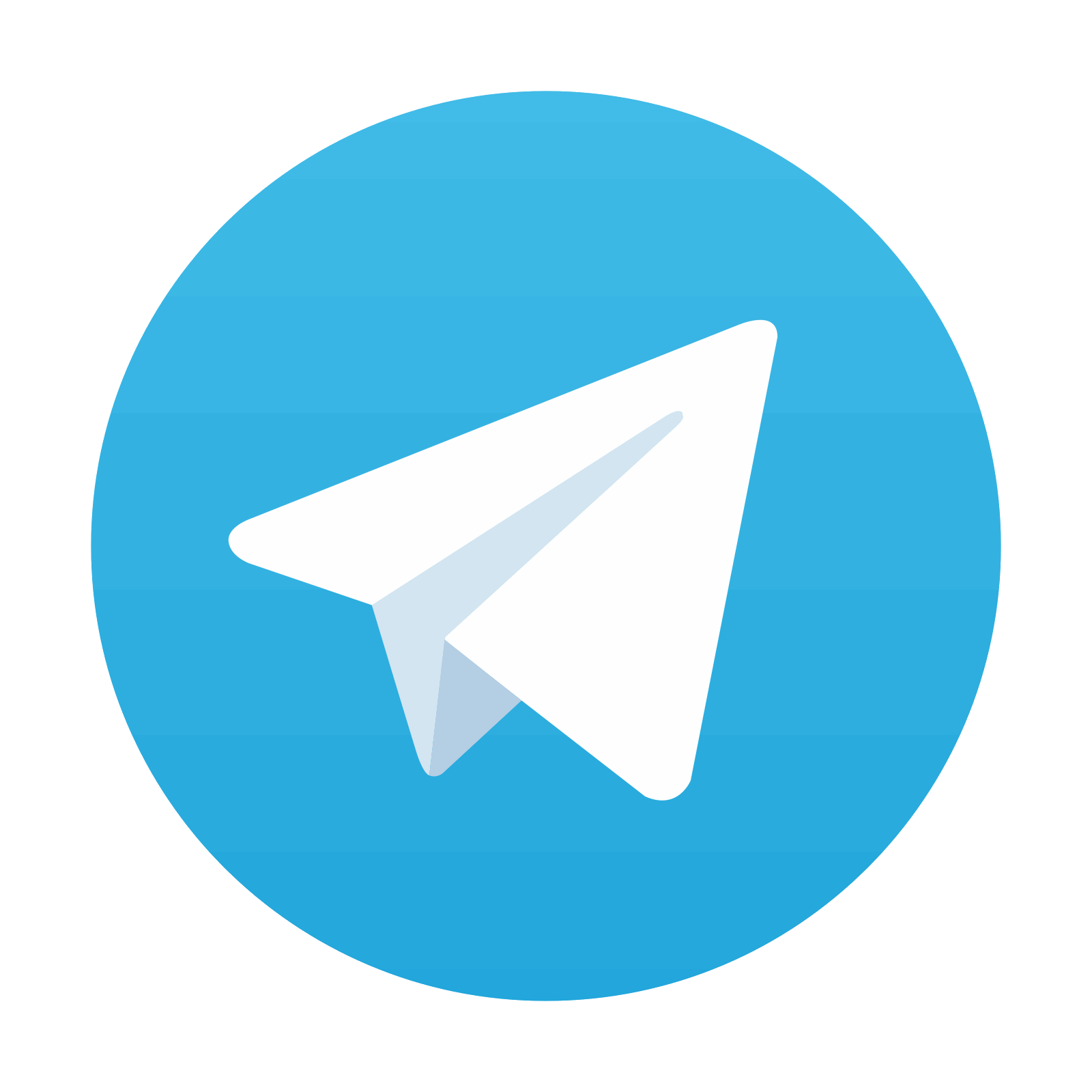
Stay updated, free articles. Join our Telegram channel

Full access? Get Clinical Tree
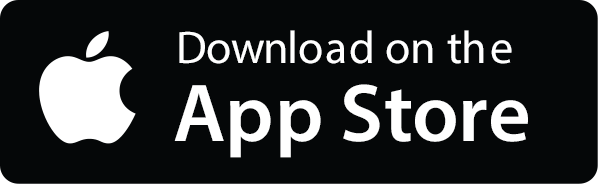
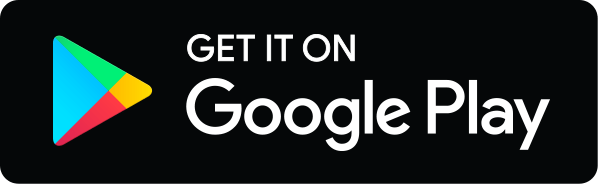