

Explain procedure to patient
Position patient in
Supine position with backrest up to 60 degrees
Lateral position at 30 or 90 degrees
CVP and PA pressures are falsely increased in Trendelenburg and should not be used10
Allow 5 to 15 minutes for pressure stabilization after position change depending on the patients underlying LV function. No specific recommendations are available for required stabilization after prone positioning; measurements have been performed 20 to 60 minutes after repositioning.
Reference and zero the pressure-transducer system
Locate the reference point
Supine: line bisecting fourth ICS at the sternum and one-half anteroposterior diameter
30-degree lateral (right and left): 1/2 distance from left sternal border to surface of bed
90-degree lateral (right): fourth ICS at the midsternum
90-degree lateral (left): fourth ICS left parasternal border
Prone: line bisecting fourth ICS at the sternum and one-half anteroposterior diameter or MAL
Level the air-fluid interface with the reference level (use either the in-line stopcock or the stopcock on the top of the transducer)
Remove the cap from the stopcock using aseptic technique
Turn stopcock “off” to the patient and “open” to air
Activate the “Zero” button on the monitor
Close stopcock and replace cap
Reference and zero the system anytime the patient’s position changes
Check and troubleshoot the dynamic response characteristics of the system every shift, if the waveform characteristics change, or if the system has been disturbed (Fig. 21-3)
Confirm Zone 3 catheter placement11
Review anteroposterior chest radiograph to ensure catheter is below LA (LA is ˜3 cm below the carina.
During wedging the PA waveform should (1) flatten into a characteristic atrial waveform (distinct a and v waves may not be discernible), (2) immediately return to a PA configuration with balloon deflation, and (3) PAOP < mean PA in absence of large V wave.
PAEDP-PAOP gradient > 4 mm Hg (may indicate Zone 1 or 2 placement).
Identify end-expiratory waveform
Determine pressures using analog (graphic) tracing
Record end-expiratory pressures
If digital data are the only available method, record the PAOP using the following:
Controlled mechanical ventilation: diastolic mode (lowest pressure)
Assisted ventilation: digital mean
Spontaneous ventilation: systolic mode (highest pressure)
With active exhalation (suspect if respiratory-induced fluctuation in PAOP is greater than 10-15 mm Hg) read the PAOP at the midpoint between the end-expiratory peak and the end-inspiratory nadir (Fig. 21-5)
With inverse-ratio ventilation use of the airway pressure waveform may help identify the end-expiratory phase and consideration should be given to correcting for PEEP or auto-PEEP.
With airway pressure release ventilation (APRV), the PAOP should be measured at the end of the positive pressure plateau, which can be observed on the ventilator and is the point immediately before the release of airway pressure and the initiation of inspiration.12
Evaluate pressures for normal fluctuation and trends
PAS: 4-7 mm Hg
PA mean: 4-5 mm Hg
PAEDP: 4-7 mm Hg
PAOP: 4 mm Hg
Improve accuracy of PAOP as an indicator of LAP with high levels of PEEP (>10 cm H2O)
Position catheter tip dependent to the LA (Zone 3—See step 6) or position patient so catheter tip is below LA (e.g., if catheter tip is in right PA, positioning the patient in right lateral position places the tip below the LA). Use angle-specific reference.
Analyze the pulmonary capillary occlusion blood. This confirms correct wedging but does not confirm that PAOP is an accurate indicator of LAP.
Estimate effect of increased transmural pressure on PAOP. Subtract ½ applied PEEP (1 cm H2O = 0.73 mm Hg) from measured PAOP.13 Example: 15 cm H2O PEEP; measured PAOP = 18 mm Hg:
15 cm H2O × 0.73 = 11.1 mm Hg
18 mm Hg − ½(11.1 mm Hg)
Estimated PAOP = 12.4 mm Hg
This is the largest pressure correction possible. Decreased compliance may lessen the effect; for example, with ARDS only 1/3 of the PEEP may be transmitted to the pleural space.14
Suspect non-zone 3 placement if with an increase in PEEP, the PAOP increases greater than ½ the applied PEEP increment (i.e., PEEP increased by 5 cm H2O (3.7 mm Hg), and PAOP increases greater than 1.8 mm Hg (3.7 mm Hg/2 = 1.8 mm Hg).

Wash hands
Gather supplies: bag of intravenous normal saline, pressure monitoring kit, 10 cc luer-lock syringe, and pressure bag with self-venting gauge
Prime pressure monitoring system to remove all air
Remove pressure monitoring kit from package, open blood salvage reservoir, tighten connections, close roller clamp, turn stopcock OFF to patient (off toward distal end), and remove vented (white) stopcock cap
Remove IV port cover and pressure monitoring line spike cover
Invert IV bag to orient bag upside down and using sterile technique, insert spike into IV bag
Leave the spiked bag upside down, open roller clamp, and simultaneously pull (activate) fast-flush device (pigtail) continuously while gently squeezing to apply pressure to IV bag to slowly clear air from IV bag and drip chamber. Completely fill the drip chamber with IV fluid.
Turn IV bag upright once fluid is advanced sufficiently past the drip chamber
Apply gentle pressure to the IV bag (or hang the bag ˜30 in. above distal end of tubing) and pull fast-flush device, advance fluid, priming the stopcock
Orient fluid so that air will be completely removed by the advancing fluid (tilt distal end of reservoir upright at 45° angle)
Pull (activate) fast-flush device while holding blood reservoir at angle, continuing to flush until the entire line is primed
Close blood reservoir, advancing all reservoir fluid through line
Perform Rocket Flush (Do not perform rocket flush if pressure line is attached to patient.)
Turn stopcock off to distal end of catheter (“off to patient”)
Attach a 10-mL syringe to the stopcock near the transducer using sterile technique and slowly withdraw IV fluid to fill syringe
Turn stopcock off to transducer (“off to monitor”)
Flush line quickly with 10 mL NS from syringe to remove any remaining air bubbles; avoid instilling any air into the line
Turn stopcock off to port and remove syringe
Cap stopcock using sterile technique with solid blue cap
Place IV bag into self-venting pressure bag and inflate to reach 250 to 300 mm Hg and recheck for air in line
Inspect line, remove any remaining air by flushing line using Rocket Flush as indicated by the dynamic response of the system (goal—adequate or optimal system)23
Table 21-1 ▪ CR-BSI CONTROL | ||||||||||||||||||||||||||
---|---|---|---|---|---|---|---|---|---|---|---|---|---|---|---|---|---|---|---|---|---|---|---|---|---|---|
|

Table 21-2 ▪ RISK FACTORS AND ACTIONS TO PREVENT COMPLICATIONS ASSOCIATED WITH ARTERIAL CATHETERIZATION | ||||
---|---|---|---|---|
|
Reflection occurs when flow is impeded (i.e., when low-resistance arteries terminate in high-resistance vessels) and the pressure wave is reflected in a retrograde (backward) fashion. This retrograde pressure wave combines with the antegrade (forward) pressure pulse, and the arterial pressure is augmented.
a derivation of Ohm’s law (pressure = flow × resistance), if resistance remains constant, there is a direct relationship between pressure and flow. However, clinically, resistance is seldom constant. Thus, BP may appear adequate while flow is decreased, or conversely BP may be low although perfusion remains adequate. Algorithms and evidence-based guidelines are available to guide assessment of the physiological and technical factors that affect direct and indirect BP measurements and outline the correct performance and interpretation of these BP measurements.22,23,97,98


CVP. An increased CVP is usually an indication of later stages of LV failure, although the CVP may remain normal even in the presence of high PA pressures and pulmonary edema.

is that there was no significant difference in outcomes between patients managed with a PA catheter or other flow measuring device and those who did not have a flow measuring device, nor was there a difference in outcomes between patients managed with a PA catheter versus another flow measuring device.156 In another study of patients with shock or ARDS there was no difference in 14- or 28-day mortality in patients with a PA catheter compared to those who received standard care without a PA catheter.157 The Evaluation Study of Congestive Heart Failure and Pulmonary Artery Catheter Effectiveness (ESCAPE) evaluated the effect in therapy guided by clinical presentation only versus therapy guided by PAC indices in 433 patients with severe, acute, or chronic HF.158 The targets were a resolution of pulmonary congestion and for the PA catheter group a PAOP < 15 mm Hg and an RAP < 8 mm Hg. Results indicated that there was no significant difference between groups in days alive out of the hospital within the first 6 months, 6-month mortality, or the number of days hospitalized. Patients in the PA catheter group did have a significantly higher time-trade-off than those in the control group. One possible explanation for this latter finding is the continued presence of increased PAOP and RAP (hemodynamic congestion) in the absence of clinical congestion.159 There is clinical sequelae associated with hemodynamic congestion, which may have been relieved in the PA catheter group. A limitation of this study was the exclusion of patients with the most advanced HF (e.g., patients with severe renal dysfunction, prior use of inotropes, and the use of mechanical circulatory support devices or mechanical ventilation).160,161

Pressures/Waveforms RA Pressure (2-6 mm Hg) | Mechanical Event | ECG Findings | Example |
a wave | RA systole | 80-100 milliseconds after P wave | |
x descent | RA relaxation | (Downslope of the a wave) | |
c wave | Tricuspid valve closure | After the QRS (follows the a wave by a time interval = PR) | Interpretation: RAP tracing from patient on mechanical ventilation. The RAP is a mean pressure (bisect an end expiratory waveform so that the areas above and below are equal or measure at the onset of the c wave). RAP = 15 mm Hg |
v wave | RA filling against closed tricuspid valve | Peak of the T wave | |
y descent | RA emptying with opening of tricuspid valve (onset of RV diastole) | (Downslope of the v wave) | |
PA Pressures | |||
Systolic (15-25 mm Hg) | RV ejection of blood into pulmonary vasculature | T wave (read at peak of waveform 0.08 second after onset of QRS (Determine by bisecting the wave) | Interpretation: PA pressure waveform from spontaneously breathing patient |
Diastolic (8-12 mm Hg) | |||
Mean (9-18 mm Hg) | |||
Indirect indicator of LVEDP | |||
PAOP (6-12 mm Hg) | |||
a wave | Left atrial systole | ˜200-240 milliseconds after P wave | Interpretation: PAOP tracing from spontaneously breathing patient. The PAOP is a mean pressure (bisect an end-expiratory waveform so that the areas above and below are equal). PAOP = 6 mm Hg |
x descent | Left atrial relaxation | ||
c wave | (Downslope of the a wave) | ||
v wave | Left atrial filling against closed mitral valve | TP interval | |
y descent | Left atrial emptying associated with opening of mitral valve (onset of LV diastole) | (Downslope of the v wave) | |
Bridges, E. J. (2000). Monitoring pulmonary artery pressures: Just the facts. Critical Care Nurse, 20(6), 59-80. See Appendix A for a full version of Display 21-3 with the pertinent strips at the end of Book on page 938-939. |
who may benefit from the use of a PA catheter to guide and evaluate therapy (Display 21-4).
the LA and ventricle; thus, the PAOP is used as an indirect measure of LV pressure. The assumption in using pressure as surrogate indicator of volume (preload) is that an increase in pressure indicates an increase in volume, and as described by Starling’s law of the heart, an increase in CO. However, there are several factors that limit the use of pressure as an indicator of volume. First, the relationship between pressure and volume is curvilinear, not linear; thus, an absolute change in pressure (e.g., PAOP) is not associated with an absolute change in volume. Second, any alteration in myocardial compliance may affect the pressure-volume relation and limit the usefulness of the PAOP as an indicator of left heart preload. Absolute PAOP values should be used with caution in any situation that alters myocardial compliance, such as LV dysfunction or myocardial infarction (MI) (particularly involving the posteroinferior surface of the heart).174 Third, the PAOP is affected by changes in pericardial pressure; thus, the PAOP may not accurately reflect transmural pressure. Finally, although the PAOP is useful for differential diagnosis and assessing the risk for pulmonary edema, it does not predict if a patient will respond to a
fluid bolus with an increase in SV.175 Functional hemodynamic indices address this limitation.
Table 21-3 ▪ HEMODYNAMIC CHARACTERISTICS OF VARIOUS PATHOLOGICAL CONDITIONS | |||||||||||||||||||||||||||||||||||||||||||||||||||||||||||||||||||||||||||||||||||||||||||
---|---|---|---|---|---|---|---|---|---|---|---|---|---|---|---|---|---|---|---|---|---|---|---|---|---|---|---|---|---|---|---|---|---|---|---|---|---|---|---|---|---|---|---|---|---|---|---|---|---|---|---|---|---|---|---|---|---|---|---|---|---|---|---|---|---|---|---|---|---|---|---|---|---|---|---|---|---|---|---|---|---|---|---|---|---|---|---|---|---|---|---|
|

Evaluation/guiding therapy in patients who remain hypotensive/hypoperfused after adequate volume resuscitation or standard therapy
Preoperative evaluation of patients who are candidates for cardiac transplantation
Evaluation/management of patients with pulmonary hypertension
Trauma victims: severely injured and older patients
Evaluation/management of patients with HF for the following conditions: failure of initial therapy, uncertain hemodynamic status (e.g., concomitant pulmonary disease or acute coronary syndrome and HF or uncertain volume or vascular resistance status), clinically significant hypotension or worsening renal/hepatic function, to optimize dosing of diuretics, inotropes, or vasoactive medications. Routine use for ADHF is not recommended.
![]() ▪ Figure 21-11 Venous infusion port PA catheter. (Courtesy of Baxter Healthcare Corporation, Edwards Critical Care Division, Santa Ana, California.) |

Prepare equipment (see Display 21-2 for line preparation)
Assist during insertion
Attach pressure tubing to proximal and distal ports and flush system.
Determine integrity of balloon (the provider inserting PA catheter will inflate the balloon); the balloon should be symmetric and not cover the catheter tip.
Transduce the distal lumen on monitor
Inflate balloon at provider’s direction (generally after catheter reaches RA).
Monitor oscilloscope for characteristic waveform changes (see Fig. 21-12A) and ectopy.
Record waveforms and pressures as catheter passes from RA to PAOP position.
Deflate balloon once PAOP has been obtained, and note return of characteristic PA waveform.
Secure catheter and note insertion distance.
Apply sterile occlusive dressing (see infection control guidelines—Table 21-1).
Obtain chest radiograph to confirm catheter placement.
Elevated a wave: conditions that increase resistance to LV filling
Mitral stenosis
LV failure (Fig. 21-13B)
Acutely ischemic LV
Elevated v wave: conditions that cause increased LA filling during ventricular systole
Acute mitral insufficiency (Fig. 21-13C)
Ventricular septal defect
Aortic regurgitation
Cardiac tamponade (Fig. 21-10)
Hypervolemia
Constrictive pericarditis
LV failure (Fig. 21-13B)
Mitral stenosis
Increased PVR (Fig. 21-15A)
Pulmonary hypertension
Chronic obstructive pulmonary disease
ARDS
Hypoxia
Pulmonary embolus
Increased pulmonary venous pressure
LV failure
Mitral stenosis
Increased pulmonary blood flow
Hypervolemia
Atrial and ventricular septal defects
Mitral insufficiency (Fig. 21-15B)
an understanding of the different causes of the HF. For example, in patients with diastolic dysfunction with a normal EF the increased PAOP reflects the primary disease. In contrast, in patients with systolic dysfunction with a decreased EF, the increased PAOP is secondary to a decrease in CO and the subsequent neurohormonal activation.185 Further discussion of the management of HF is presented in Chapter 24.
introduce error into pressure measurements and potentiate therapeutic mismanagement of critically ill patients.
In the lateral position, PA and PAOP can be obtained in the 30and 90-degree lateral positions, as long as an angle-specific reference is used (Display 21-1). Because some patients respond differently to position change, pressure measurements obtained in the flat, supine position should be compared with those obtained with backrest elevation or lateral position before assuming no difference.
Table 21-4 ▪ HEMODYNAMIC PROFILES WITH PULMONARY HYPERTENSION | |||||||||||||||||||||||||||||||||||||||||||||||||||||||||||||||||||||||||
---|---|---|---|---|---|---|---|---|---|---|---|---|---|---|---|---|---|---|---|---|---|---|---|---|---|---|---|---|---|---|---|---|---|---|---|---|---|---|---|---|---|---|---|---|---|---|---|---|---|---|---|---|---|---|---|---|---|---|---|---|---|---|---|---|---|---|---|---|---|---|---|---|---|
|

Verify the order to remove the catheter
Assemble necessary equipment
Document on the flow sheet the ECG rhythm and vital signs before initiating the procedure
Explain the procedure to the patient
Transfer IV infusions from PA catheter ports to side port of introducer or discontinue IV solutions if appropriate
Ensure that the patient remains in hemodynamically stable condition after transfer of infusions to side port
Turn off any remaining infusions to distal and proximal ports
Ensure that the balloon is deflated by lining up the red lines on the balloon port, drawing back on the syringe, and then discontinuing the syringe
Place the patients supine and turn the patient’s head away from the insertion site
Open the sterile obturator/introducer cap, ensuring that sterility of the cap is maintained
Put on examination gloves
If the catheter dressing is nonocclusive or covers the introducer, after putting on a mask, remove the dressing
Instruct the patients to inspire deeply and hold their breath (or apply positive pressure breath on ventilator) during withdrawal of the catheter
Unlock the catheter shield from the introducer
While securing the introducer with nondominant hand, withdraw the catheter with dominant hand, using a constant steady continuous motion
Observe the ECG continuously during withdrawal of the catheter
If any resistance is met, do not continue to remove the catheter, and notify the physician immediately
Once the PA catheter has been removed, don sterile gloves and insert a sterile adaptor cap into the diaphragm site of the introducer
If necessary, reapply a sterile dressing to the catheter site according to policy
Elevate head of bed and return patient to position of comfort
Examine balloon and catheter to ensure that they are intact. If they are not intact, notify the physician immediately
Document on flow sheet the patient’s response to procedure, including vital signs and ECG rhythm
Table 21-5 ▪ TROUBLESHOOTING THE PA CATHETER AND MEASUREMENT PROBLEMS | ||||||||||||||||||||||||||||||||||||||||||||||||||||||||||||||||||||||||||||||||||||||||||||||||||||||||||||||||||||||||||||||||||||||
---|---|---|---|---|---|---|---|---|---|---|---|---|---|---|---|---|---|---|---|---|---|---|---|---|---|---|---|---|---|---|---|---|---|---|---|---|---|---|---|---|---|---|---|---|---|---|---|---|---|---|---|---|---|---|---|---|---|---|---|---|---|---|---|---|---|---|---|---|---|---|---|---|---|---|---|---|---|---|---|---|---|---|---|---|---|---|---|---|---|---|---|---|---|---|---|---|---|---|---|---|---|---|---|---|---|---|---|---|---|---|---|---|---|---|---|---|---|---|---|---|---|---|---|---|---|---|---|---|---|---|---|---|---|---|
|
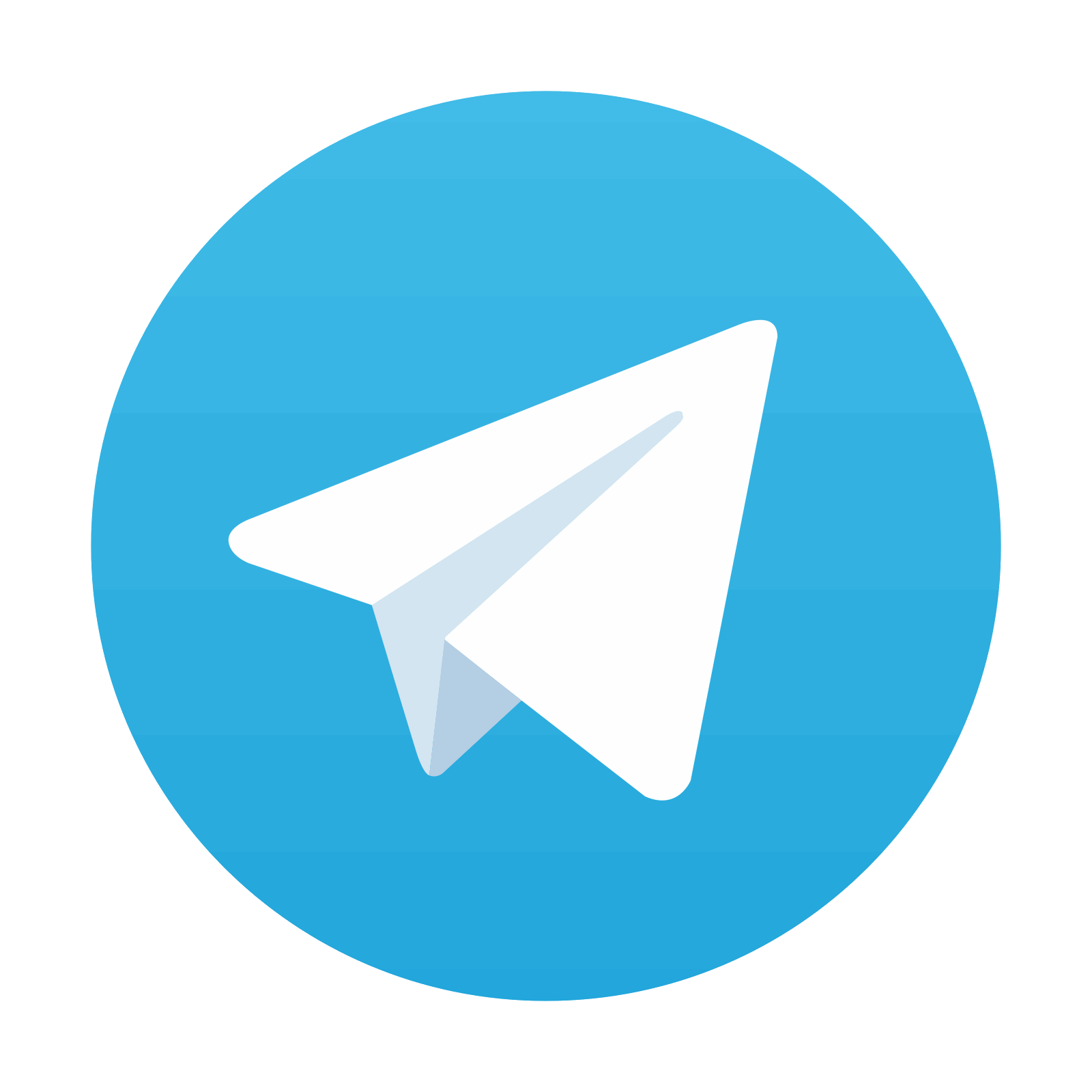
Stay updated, free articles. Join our Telegram channel

Full access? Get Clinical Tree
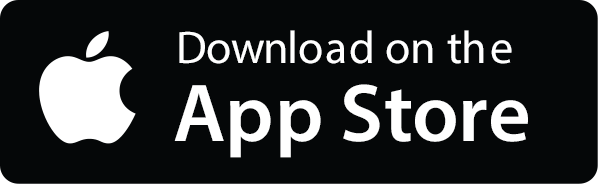
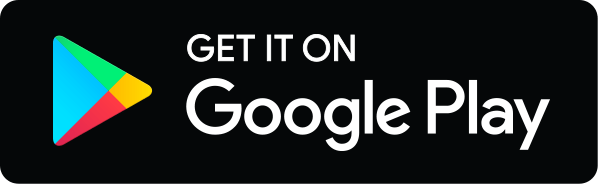