Echocardiography
Peter J. Cawley
Echocardiography (echo) is ultrasound technology as applied to imaging the heart and the associated great vessels.1 As cardiac catheterization and invasive angiography transformed our understanding of the structure and function of the heart, echo allowed clinicians the same opportunities noninvasively. Our clinical experience utilizing echo for patient care is vast and spans many decades. Echo is one of the most widely used imaging modalities today within cardiology because of the following attributes: safe, fast, lacks exposure to ionizing radiation, noninvasive, and portable.
In the United States, the acquisition of echo images is most commonly performed by sonographers (individuals who have completed specialized training programs in ultrasound), echocardiographers (cardiologists with training in echo), or cardiovascular anesthesiologists. As the field has expanded, other physicians including those who practice emergency medicine, primary care, or critical care have received training in image acquisition.
This chapter will focus on three general topics: (1) principles and techniques of echo, (2) echo examination, and (3) special applications of echo.

Sound Waves
Ultrasound is sound with a frequency above 20,000 Hz (cycles per second) or 20 kHz. This sound is outside of the range of the human ear. Diagnostic ultrasound for cardiovascular imaging uses frequencies ranging from 2 to 30 MHz: adult transthoracic frequencies range from 2 to 4 MHz, transesophageal frequencies from 5 to 7 MHz, and intravascular ultrasound frequencies (coronary artery) from 20 to 30 MHz.
Sound waves are generated at the transducer, the hand-held probe that is applied to the body part of interest. Today, transducers contain a piezoelectric crystal, which when applied to an electric current, will cause the crystal to deform. This deformation results in the generation of a sound wave, which is transmitted to the body. Returning sound waves can also deform the crystal and thus be detected by the transducer.2 The transducer receives sound waves that are generated from the interaction of the transmitted sound waves and the tissues of the body. The transducer generates and receives reflected sound waves. Interestingly, the time the transducer is in receiver mode (i.e., listening mode) is far greater than when in transmitting mode (i.e., generating sound waves) with one exception—continuous wave Doppler, which will be explained further (See Doppler).
Sound moves through media in the form of waves and may interact with the tissues in different ways. This chapter presents two of the more common interactions, reflection and attenuation. Reflection occurs when a transmitted sound wave interacts with tissues of different density within the body. For example, there is a different density of the blood than of the myocardium. The boundary between the two tissues causes reflection of the transmitted sound wave, which is then detected by the transducer. Depending upon the time it took for the transducer to emit the sound wave and detect the reflected sound wave, it will place that object at a certain distance (depth) on the image.2
Attenuation is degradation of the sound wave as it propagates from the transducer. Sound waves pass through tissues and attenuate with greater distances from the transducer. This attenuation results in a finite ability of the transducer to capture reflected sound waves from greater depths. The greater the distance the transducer is from the heart, the greater the number of sound waves that will be attenuated, and the result will be poor image quality. Examples of sound wave attenuation for echo caused by greater depth (distance) include obesity and breast tissue. But depth is not the only cause of sound wave attenuation in echo. Bone and air also result in considerable attenuation. Because the heart is surrounded by the sternum and ribs, imaging can be performed only in the rib spaces. In patients with smaller ribs spaces, imaging can be particularly challenging. Because the heart is in close proximity to the lungs and trachea, these structures must also be avoided. In patients with enlarged lung spaces (i.e., chronic obstructive pulmonary disease), imaging can be difficult. Pneumomediastinum, pneumothorax, and subcutaneous air will all result in signal attenuation.
Experienced echocardiographers and sonographers should possess a thorough understanding of the impact of image artifacts. Inability to recognize artifacts could lead to false interpretation, some with significant consequences. Ultrasound can cause several different types of artifacts and an explanation of each is beyond the scope of this chapter. To reduce misdiagnosing artifacts, abnormalities should be visualized in more than one imaging window, thus reducing the likelihood of false interpretation.
M-Mode
M-mode (motion) echo displays a narrow ultrasound beam of information within the heart along the y-axis (vertical axis) and displays it according to time on the x-axis (horizontal axis). Heart structures are displayed with respect to motion and time. M-mode echo provides high temporal resolution and provides information regarding both the structure and function of the heart. M-mode echo predated the existence of two-dimensional (2-D) echo and although it is not as commonly used as in the past, M-mode can still be useful to describe motion of structures of the heart with respect to the cardiac cycle (Fig. 13-1).
2-D
2-D echo was developed from similar concepts of M-mode. Because M-mode only allowed a very narrow and focused area of
interrogation of the heart, it was limited. 2-D echo provided a wider area investigation into the structure and function of the heart within a 90-degree scanning sector.1 Thus, 2-D echo provides a more complete investigation of the entire structure and function of the heart (Figs. 13-2, 13-3, 13-4, 13-5 and 13-6).
interrogation of the heart, it was limited. 2-D echo provided a wider area investigation into the structure and function of the heart within a 90-degree scanning sector.1 Thus, 2-D echo provides a more complete investigation of the entire structure and function of the heart (Figs. 13-2, 13-3, 13-4, 13-5 and 13-6).
Doppler
Doppler affords clinicians a powerful and integral tool for assessing heart function. This is based on the Doppler principle, first described by Christian Johann Doppler in the 19th century. It describes the change in reflected sound wave frequency compared with the transmitted sound wave frequencies generated from the transducer. These sound waves are reflected off of moving red blood cells. This change in frequency (transmitted to reflected sound waves) is related to the velocity of moving red blood cells through the heart, which can be used to describe the hemodynamics of blood flow through the heart.
One important feature of Doppler interrogation is the angle of the transmitted frequency as compared to blood flow. To achieve the most accurate estimate of the velocity of blood flow, the angle of interrogation (angle of the transmitted sound wave) should be parallel to blood flow. As the angle increases, so does the error, which results in underestimation (never overestimation) of blood flow velocity. Because air (lung) and bones (ribs and sternum) limit the number of imaging spaces that can be used to interrogate blood flow through the heart, Doppler interrogation should be performed from multiple locations to minimize this error. The highest velocity obtained should be interpreted then as the sound wave most parallel to blood flow.1, 2, 3
There are three types of Doppler techniques: pulse wave, continuous wave, and color. Pulse wave Doppler is used for the assessment of blood flow at specific locations and is useful for velocities less than 2 m/s (Fig. 13-7). Continuous wave Doppler is used for assessing velocities along the entire pathway of the sound wave and is used for velocities up to 8 m/s (Figs. 13-8 and 13-9). Continuous wave Doppler as its name suggests is continuously transmitting and receiving sound waves using separate crystals. This technique is unlike all other echo techniques, such as 2-D echo, pulse wave Doppler, and color Doppler, which all use the same crystal to transmit and receive sound waves and predominantly spend the majority of time receiving sound waves.
Color Doppler is a pulse wave technique in which multiple points in a specified sector are sampled. Depending upon the direction and turbulence of blood flow, a color is encoded upon a 2-D image (Fig. 13-10). This technique is useful for visualizing the presence of blood flow, the presence of turbulent blood flow, and shunts.

Patient Preparation
Imaging is most ideally performed on special examination beds that have a removable section of the mattress to allow placement of the imaging transducer along the patient’s left side. This simple feature improves the quality of the examination. However, there are circumstances where the patient cannot be transported safely to the echo laboratory (i.e., ICU level of care, emergency) and in that case, the ultrasound machine can be brought to the patient’s bedside. The portability of echo is unique among cardiovascular imaging modalities.
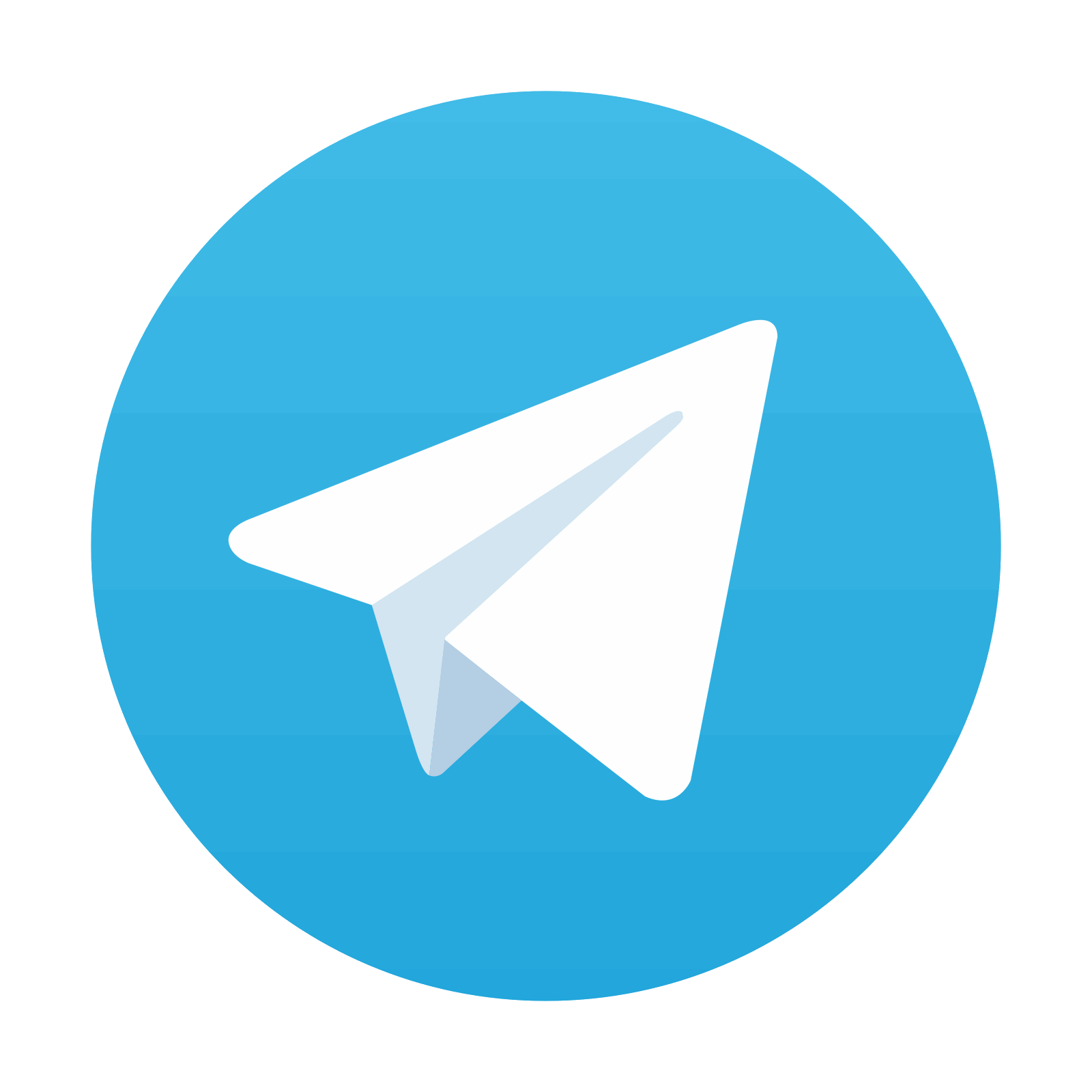
Stay updated, free articles. Join our Telegram channel

Full access? Get Clinical Tree
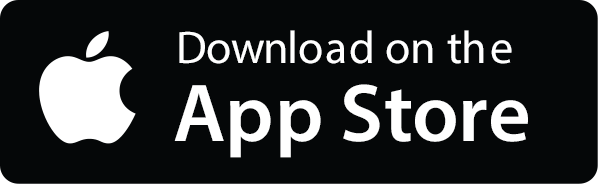
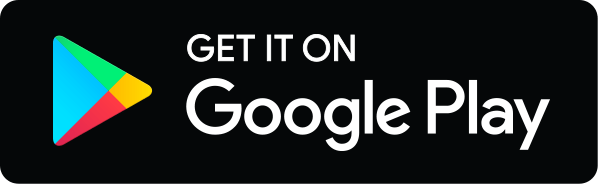